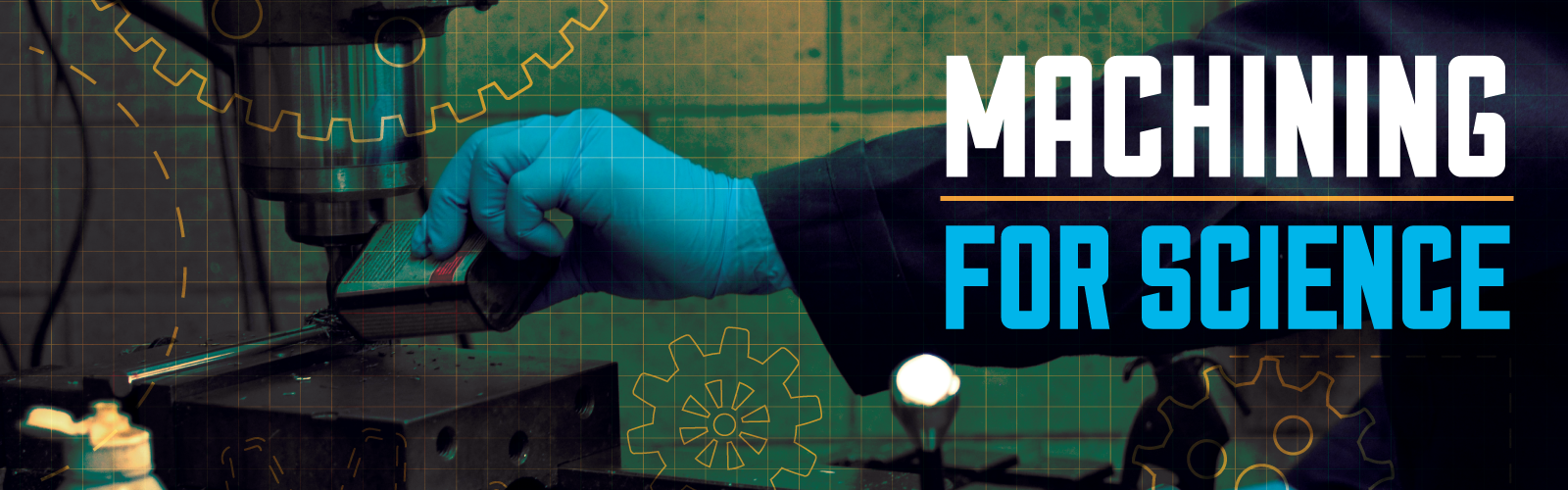
Machining for science
In the basement of Building 13 at the National Institutes of Health’s Office of Research Facilities, George Dold and his crew of engineers and machinists in the Section on Instrumentation strive daily to meet the needs of investigators with unconventional demands.
Their group is one of two on the NIH campus that build devices commercial lab manufacturers don’t, products ranging from metal-free pneumatic flavor droppers to eight-armed radial mouse mazes.
The crew is fond of a clip from the 1999 animated adaptation of the comic strip “Dilbert” in which the underappreciated office drone recalls a childhood visit to a doctor.
“It’s worse than I’d feared. I’m afraid your son has the knack,” the doctor tells Dilbert’s mother. “It’s a rare condition characterized by an extreme intuition about all things mechanical and electrical, and utter social ineptitude.”
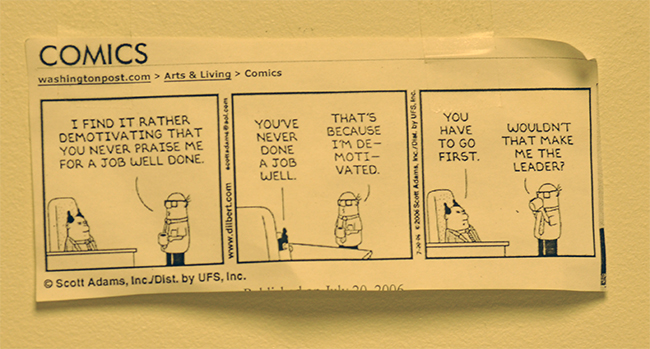
of the comic strip Dilbert. This panel is posted on the wall of their lab.
The latter half of the characterization may be self-deprecation, but the former rings true — at age 7, Dold couldn’t keep his hands off tools in his father’s lab, which was at the National Institutes of Health.
“I’ve always been a tinkerer,” Dold said. “I also know how to fabricate quite a bit. Don’t ask the machinists about that, though, because they’ll say otherwise.”
The Section on Instrumentation has its roots in a technical support group founded in the 1950s by the intramural divisions of the National Institute of Mental Health, National Institute of Neurological Disorders and Stroke, and National Institute of Child Health and Human Development to share developments among the institutes. As computer use increased in the following decades, the group shifted to provide support for NIH labs through engineering, machine shop work and information technology services. When the IT work was spun off to another group, the engineering and machine work subgroups became the Section on Instrumentation.
When Tom Talbot, a mechanical engineer in the section, began working at the NIH in 1975, one of the primary tasks of the more than two dozen machinists with a different group in Building 13 was automating the process of inserting cotton into freshly washed test tubes.
“All of that stuff went away with disposable glassware and what have you,” Talbot said, “so those skills had to evolve or else go away, and to a large degree they went away.”
The change in skill sets corresponded to a shrinking staff. Soon after Talbot arrived, the machinists were cut from two full-time shifts to one, which numbered about 35 mechanical, electrical and chemical engineers and 15 machinists, by Talbot’s count.
Today, Dold’s group includes four engineers and four machinists, or technicians, each with skills uniquely suited to the projects they handle.
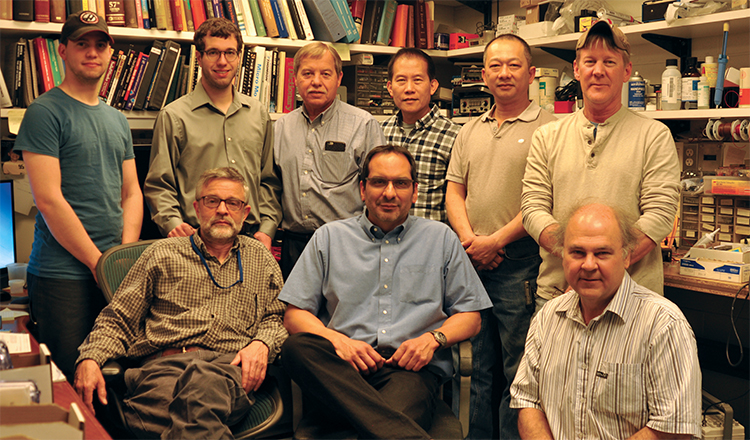
each year. Pictured in back are, from left, Daniel Yochelson, William Bennett, Tom Talbot, Danny Trang, Phuoc Pham and Daryl Bandy; in front
are David Ide, George Dole and Bruce Pritchard.
Taste tests and mouse mazes
David Ide, a biomedical engineering technician, specializes in electrophysiology devices, such as microdrives that help move electrodes into the skulls of rodents and primates. He worked as a machinist at a small business in Maryland for 15 years before coming to the Section on Instrumentation 22 years ago.
“The specifics of the job change enormously; it continues to change,” Ide said. “We used to make electrophoresis equipment, but no more. It’s all commercially available.
“The guy I replaced said when he came to NIH they were sticking electrodes in frog legs. By the time he left, they were sticking electrodes in brain cells.”
Another machinist, Daryl Bandy, and William Bennett, an engineer, operate the section’s four 3-D printers. The printers are essential for constructing plastic devices, ranging from custom multiangle electrode holders to tables with trapdoors to conceal and reveal objects, that go into MRI machines for behavioral studies.
Talbot is an NIH lifer. After completing his master’s degree in mechanical engineering, he took a position studying hemodynamics at the National Heart, Lung and Blood Institute; he later moved to that institute’s clinical center to help develop surgical tools for open-heart research. He followed almost two decades there with a 10-year stint at the institute’s Electron Microscopy Core before joining Dold’s group, where he tackles electromechanical work.

Bandy has helped build is a waveguide
to help send signals to and from
the control room.
In 2010, Talbot helped construct an MRI-friendly contraption of valves and tubes, a gustometer for a joint study with researchers at the National Institute of Mental Health and National Institute of Diabetes and Digestive and Kidney Diseases.
“(The researchers) wanted a way to drop different tastes onto a patient’s tongue while they’re in the MRI,” Talbot said. “Then they would look at different areas of the brain that would light up when the patients tasted salt, bitter, sweet, sour, et cetera.”
The design for the gustometer, Talbot said, was copied and picked up in research hospitals across the country for studies on obesity. More than flattery, the section workers seem to take this imitation as a point of pride.
A more recent addition to the group, Bruce Pritchard, worked as an electrical engineer at Hewlett Packard in Oregon for nearly 30 years before a terminal cancer diagnosis brought him to Bethesda for a clinical trial at the NIH in 2011. During one of his many hours-long visits to the campus, Pritchard found his way to the Section on Instrumentation, where he struck up a friendship with his fellow tinkerers.
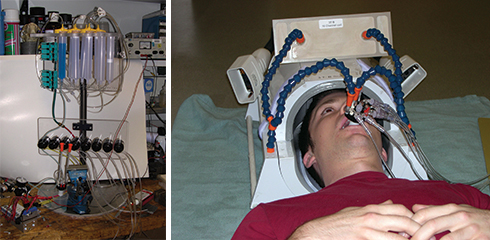
which are used for a variety of taste test research, in 2009.
Right: The gustometers are designed to fit in an MRI machine, here demonstrated by Mark Reddish, a former postbaccalaureate worker in the lab. Tom Talbot recently updated the fluid regulating manifold and mouthpiece to a 3-D printed design.
With his cancer in remission, Pritchard returned to the West Coast but stayed in touch with Dold. When a position in the section opened up, he applied, and he has been with the group for four years. At HP, Pritchard had designed equipment to pick up biophysical signals, and he hoped to continue that work at the Section on Instrumentation.
“I was looking forward to getting involved in amplifier design, and, lo and behold, I get here and there’s very little need for it, but it’s because the industry has stepped up to the plate,” Pritchard said. “Fortunately, there’s plenty of other fascinating stuff, and it’s more (cutting edge) than what I came here thinking I was going to be able to contribute to.”

Every few years, the group is tasked with an enormous project that draws on their collective skills and human power. A recent years-long project involved the construction of a laboratory that met the latest guidelines for good manufacturing practices, a facility with lead-lined radioactive ovens, or hot cells, in which robots forge the radionuclides essential for the countless PET scans done at the institute.
“They had the hot cells, (but) we basically had to integrate everything inside of them,” Dold said.
On a smaller scale, Dold works with mouse mazes run by the systems engineering software LabVIEW. The legions of scientists involved in behavioral research at the National Institute of Mental Health regularly request the mazes, and Dold recently built one with eight branched pathways, each with automated doors and mouse tracking systems.
“The maze was 4, 5 feet in diameter,” Dold said. “But we made it where you could take it apart pretty easily and robustly, and put it together. That was a fun project.”
Sides of a coin
Tom Pohida’s engineering group, the Signal Processing and Instrumentation Section, or SPIS, at the NIH fulfills a similar, complementary role to Dold’s team, whose electromotive machinations are primarily used by researchers at the National Institute of Mental Health, National Institute of Neurological Disorders and Strokes, and National Institute of Child Health and Human Development. The Pohida group supports the whole NIH intramural research program when the needs of researchers grow beyond the reach of commercially available lab equipment and software or established laboratory and clinical methodologies.
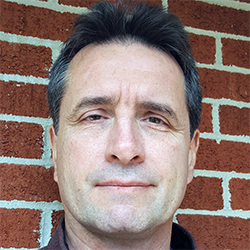
Pohida’s group of seven full-time engineers, with 10 to 15 interns throughout the year, was part of the NIH team that helped invent laser capture microdissection in the mid-’90s. The technique allows scientists to isolate specific cell types from tissue samples, an important purifiation step that precedes molecular analyses.
“That was fully prototyped in-house, validated, patented and licensed. It’s a gold standard in the tissue microdissection field,” Pohida said. “But then, more recently, we developed a microdissection new method.”
The first method is microscope-based and involves a user selectively firing a laser to melt and bond a dyed light-absorbing film to the cells of interest. This allows the targeted cell populations to be removed from the slide by pulling off the film when it’s cooled. The new method, targeted activated microdissection, uses cell- or organelle-specific staining to absorb diffuse light to melt the clear film only at the desired targets. This multiyear, multi-institute technology development evolution is typical of the group’s interdisciplinary projects, which are collaborative efforts that span 18 NIH institutes and centers.
“Our projects at NIH are rarely a one-off, a one-time effort,” Pohida said. “We’ll place an instrument in (a researcher’s) lab, and then their ideas change, methods evolve, instruments need to be modified, and then there’s a second- or a third-generation system to design and build.”
In one of Pohida’s recent collaborations, his group and Nicole Morgan, the acting chief of the Trans-NIH Shared Resource on Biomedical Engineering and Physical Systems, developed a novel micron-sized scaffolding device that acts as a bioreactor for the NIH researcher Michael Gottesman, who studies mechanisms of multidrug resistance in cancer.
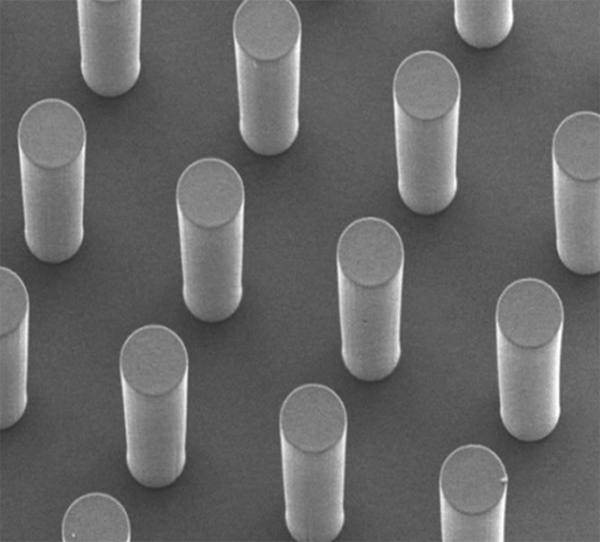
at NIH’s Center for Information Technology designed for Michael Gottesman are small enough for cancer cells
to grow around, measuring approximately 240 microns tall and 82 microns wide.
“This is a problem we’ve been working on since the early 1980s, and it persists,” Gottesman said. “We get better and better at developing drugs, and almost all of them fail because of resistance.”
One obstacle to studying cancer cells is the surface the cells are grown on; many cancer cells have a strikingly different pattern of gene expression in a two-dimensional monolayer in a petri dish than they would exhibit in a human body. To better replicate that environment — for which mice make a poor proxy — and the blood vessels the cells use for oxygen delivery, Gottesman and his colleagues turned to Pohida and Morgan for a micro-fabricated polymeric vessel mimetic.
The device, which consists of micron-size pillars the cells can grow around, is essentially an artificial capillary system that delivers oxygen directly to the tumor cells rather than relying on diffusion through a medium.
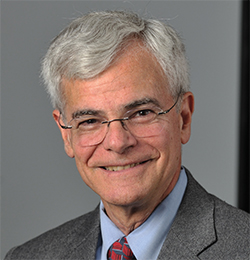
Gottesman and his team are still at the early stages of characterizing the device.
“The recent data suggest that the growth of the cells and the gene expression of the cells is very different when they’re grown in this new bioreactor,” Gottesman said, “so we’re hoping that that reflects changes in how they respond to drugs and mechanisms of resistance.”
Fabricators, forensics and charters of freedom
The work done by Dold and Pohida’s groups is not uncommon outside the NIH. Machine shops at public and private research universities fabricate equipment to fit researchers’ needs, with some supporting single departments and others serving entire schools within a university.
The Section on Instrumentation and SPIS also aren’t the only resources for National Institutes of Health scientists who require the help of mechanically skilled hands. When researchers need a piece of lab equipment modified, they often call upon Howard Metger and Robert Clary in the Office of Research Facilities.
Metger and Clary improve existing lab equipment, including centrifuges, microscopes and incubators, and design devices that can locate eyeballs, separate blood and support knees.
After a four-year apprenticeship at Landis Machine Company in the 1980s and local work with a trucking company, Metger began his federal government career as a machinist at the Naval Ordnance Laboratory in White Oak, Maryland, in 1987.
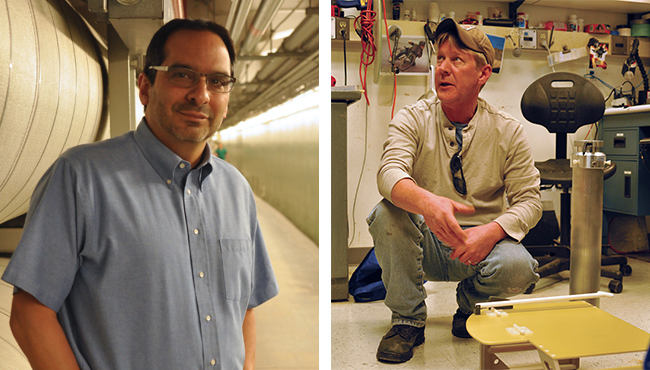
engineering and a master’s degree in mechanical engineering.
Right: One of the MRI-friendly devices Daryl Bandy has helped construct with 3-D printing is a plastic table with a door
that allows a user to reveal and conceal objects. The effect this has on areas of the brain
can then be observed by researchers monitoring the MRI output.
In 2004, Metger began working at the Office of Research Services’ Mechanical Instrumentation Design and Fabrication Branch, which was transferred to the Office of Research Facilities last fall due to a new funding model. Clary, who began working at the National Institute of Standards and Technology in a co-op position in 1990 after graduating from Carroll County Vocational Technical School in Westminster, Maryland, moved to the ORS in 2006.
During his time at the NIST, Clary helped develop a standard calibration tool for the ballistics reference databases used by all 50 states and the FBI, known as the Integrated Ballistic Identification System.
As a bullet is fired from a gun, the gun’s chamber leaves a serial number-like series of grooves on the bullet. When a bullet is found at a crime scene, those grooves can be run through a ballistics reference database, which will assign a score for how likely a match the grooves are to a gun in the system.
“When you take those (scores) to a court of law and you have a defense attorney ask how good it is, you need a reference material to compare individual systems,” Clary said. “The only way to do that was to take one bullet and send it around to all 50 states and to the FBI and have them measure it, and hopefully all of their scores come up the same. But a better way to do it would be if we had 50 copies of the exact same bullet.”
To cut those identical reference grooves into fifty-plus bullets, Clary and his colleagues at the NIST used a lathe equipped with a diamond crystal sharpened to a near-atomic point. They then sent the bullets to each ballistics center to get their tools — what Clary calls “a computer with a microscope” — on the same page.
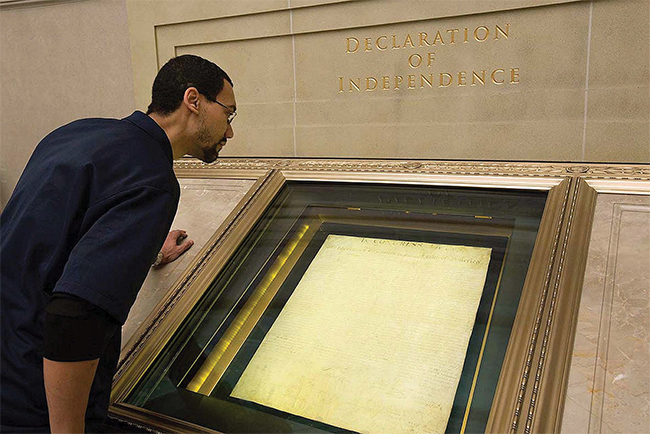
and Robert Clary helped construct while working at the National Institute of Standards and Technology.
“It didn’t solve any problems, but what it did do was it allows the court systems to lean a little heavier on the IBIS system,” Clary said. “We can’t just use the score to say a crime was committed, but it opens up avenues that are much more reliable.”
In 1999, Metger and Clary, then working together at the NIST, were contracted to help build new versions of the bronze and glass cases in the National Archives Building that preserve and display the United States Declaration of Independence, Constitution and Bill of Rights, otherwise known as the Charters of Freedom.
“I built all of the cases and document platforms and lids for them, and Bob worked on a lot of the electronics that went on the inside of the cases,” Metger said.
The documents, first encased and displayed at the National Archives in 1952, now are kept in sealed cases of glass, titanium and aluminum.
At the ORF, Clary is working on a similar project to preserve and display the charts and notes of Marshall W. Nirenberg, a researcher at the National Heart, Lung and Blood Institute who shared the 1986 Nobel Prize in physiology or medicine for discovering the molecular mechanisms responsible for translating DNA into proteins.
“The charts that he used when he was doing his research back in the day are now these Rosetta stone-type things, and the National Library of Medicine has decided that they want to encase these in some anaerobic boxes,” Clary said. “We’re not having luck with the commercially available boxes as of yet, so that’s an ongoing project.”
Some assembly required
Outside the public sector, working on equipment in molecular biology labs largely comes down to calibrating the instruments and replacing worn-out parts. Matt Helmrick is a laboratory technician at Dynex Technologies, a company mostly known for its multiplate enzyme-linked immunosorbent assay processing machines. Helmrick services the machines designed to detect the presence ofsubstances in liquid or wet samples.
“Some of the ones you don’t want to deal with (can be) in a very high-throughput lab, where the technicians come in on shifts and the machines just don’t stop,” Helmrick said. That nonstop use means the machines’ valves and tubes need to be replaced much sooner than expected. “And if (the machines) are in forensic toxicology or something, they’re covered in things you don’t want to touch.”
On the production side, Dynex’s group of fabricators oversees the assembly of each machine, testing circuit boards and tubing before sending them out to labs across the country.
For many of the fabricators, “this is the first job they got as an intern out of high school, and they ended up working here for 20 years,” Helmrick said. “I know one guy was really good at pinball machines — he joined quite a while back, and now he’s overseeing one of the departments.”
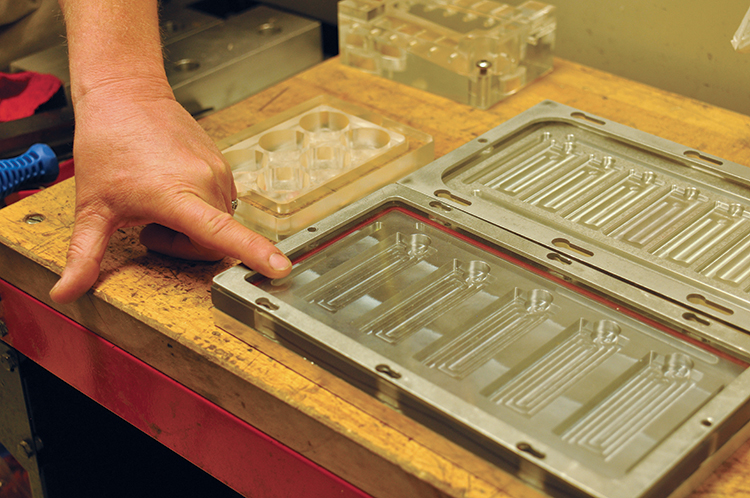
to simultaneously run dyes on multiple slides while using minimal reagents.
Helmrick began as a research scientist in San Francisco, bouncing around biotech companies and eventually winding up at Bristol–Meyers Squibb, where he worked in research and development related to genomic technology.
“Higher and higher genomic and PCR work requires a lot of back-end work, so it helps if you have some coding experience to write your own searches, format files correctly, and look for genes of interest,” he said.
The less sophisticated lab work, though — pipetting, plating and every manner of PCR-prepping — is already in the realm of automation and likely to be completely overtaken by it, Helmrick believes.
“I think lab technicians are going to get replaced by machines long before truck drivers ever have to worry about self-driving trucks,” he said.
Creativity, however, is currently beyond the purview of machines and plays a large part in the work Clary and Metger perform for researchers.
“I think, internally, I’ve changed my attitude of being a machinist to being more of a researcher’s assistant. When I first started in the trade back in the day, I was a machinist, and I still am at heart very much a machinist,” Clary said. “I like to cut precision things, I like to make things that don’t exist, but at the same time … I spend a tremendous amount of time gathering details and making suggestions.”
Despite the pace of development in biomedical technology, Dold is optimistic about the future of the Section on Instrumentation.
“I think that we’re going to have to continue to try to figure out ways in which to build equipment quicker and adapt more technology quicker,” Dold said. “I think that the 3-D–printing revolution that was supposed to keep on exponentially growing (has) tapered off, but I still think it’s going to have continued impact with us.”
Every year, Dold’s group averages around 500 projects, a number of which are lower tech and do not require tools like the 3-D printers.
“There’s still a lot of people that need simple things done, and they need it done quickly,” he said. “The jobs range from relatively low tech to very complex engineered systems with many features. We have to be able to do both ends of the spectrum.”
Mentoring over the long term
Like many groups within the NIH, the engineers in the SPIS are strongly committed to mentoring college and high school students. Through the NIH’s Pathways co-op program, Tom Pohida and his co-workers bring on about a half-dozen engineering students from local colleges for full-time work each summer, which they often continue part-time during the fall and spring semesters.
“Our engineers are outnumbered by interns in the summer,” Pohida said. “We’re able to give the students a meaningful exercise for their career development and get them to a point where they’re productive and working on research projects at NIH, developing instruments and software algorithms.”
Pohida’s group also participates in the 10-week National Institute of Biomedical Imaging and Bioengineering Summer Internship Program, which selects 16 interns between their junior and senior years of college to work on collaborative projects on the NIH campus.
The section also participates with mentor programs at local high schools that allow students to learn engineering skills during the week as a part of their curriculum.
A number of the Pathways interns stick around through their college years, Pohida said, and some are hired as federal employees after they graduate. At the moment, three of the six engineers working in the SPIS are former interns.
Sarah Anderson, a second-year medical student at Virginia Tech, worked in the SPIS through the co-op program for three years when she was an undergraduate at the University of Maryland. An electrical engineering major, Anderson began working at the SPIS the summer after her freshman year and continued through her graduation in 2017.
“I really liked how different all of the projects were and how much equipment we had,” she said. “It’s very unique in that the projects changed and you could learn very different skills that we don’t learn in our curriculum.”
A relatively new medical school, the Carilion School of Medicine and Research Institute at Virginia Tech incorporates longitudinal research projects into students’ curricula. Anderson believes her time at the SPIS made her a stronger candidate for both the application process and for choosing which lab she would work in.
“I really wanted to continue doing the same kind of work I was doing at NIH, so I talked to a lot of different people and I was able to have the practical skills where I could sell myself,” she said.
Anderson now balances her coursework with work in the lab of Alan Asbeck, who designs and builds motion-assisted exoskeletons for lifting heavy objects.
Anderson’s project in the lab involves designing low-cost circuit boards that filter and process the electrical signals produced by skeletal muscles, allowing a user or patient to control the exoskeleton. The project has additional applications for stroke patients who have suffered loss of motion and have difficult extending, flexing or bending an arm.
“The idea would be to have these sensors placed on the forearm,” Anderson said. “If you could only partially do the motion, the muscle impulses would tell the exoskeleton to actively assist you.”
Enjoy reading ASBMB Today?
Become a member to receive the print edition four times a year and the digital edition monthly.
Learn moreGet the latest from ASBMB Today
Enter your email address, and we’ll send you a weekly email with recent articles, interviews and more.
Latest in Science
Science highlights or most popular articles
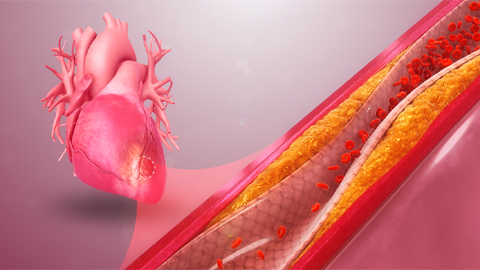
Scientists find unexpected correlation between age and HDL-C levels
In a 30-year multicenter study, researchers determined what factors predict HDL-C concentration. In their analysis, they found that HDL-C levels grew with increasing age and physical activity.
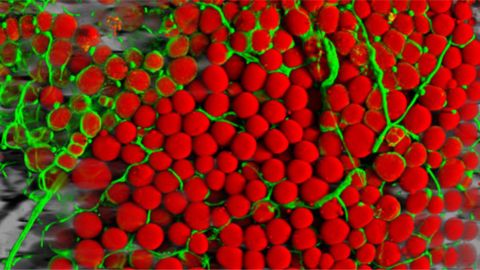
Butter, olive oil, coconut oil — what to choose?
Depending on the chain length and origin of the fat, regular fat consumption changes the specific makeup of fats in bloodstream and affect mild to severe cholesterol patterns. Read about this recent Journal of Lipid Research study.
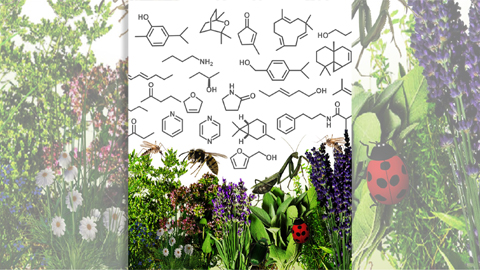
Computational tool helps scientists create novel bug sprays
Rapid discovery of mosquito repellent compounds is enabled through a novel screening platform that combines both computational modeling and functional screening.
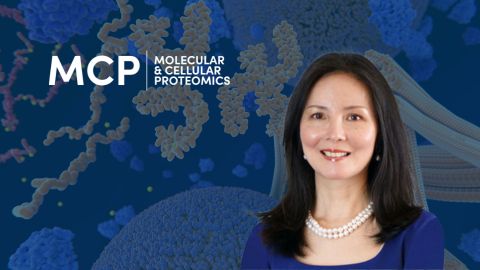
Meet Lan Huang
Molecular & Cellular Proteomics associate editor uses crosslinking mass spec to study protein–protein interactions to find novel therapeutics.
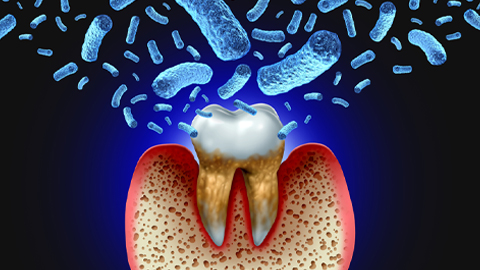
Influenza gets help from gum disease bacteria
Scientists discover that a protease from Porphyromonas gingivalis enhances viral spread. Read more about this recent Journal of Biological Chemistry paper.
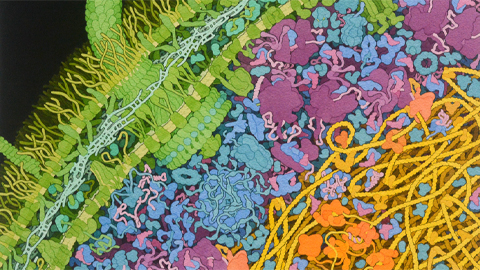
How bacteria fight back against promising antimicrobial peptide
Researchers find a mutation in E. coli that reduces its susceptibility to a potential novel antibiotic. Read more about this recent Journal of Biological Chemistry paper.