World Malaria Day
What comes to mind when you image a deadly animal? Perhaps something large and imposing, with piercing claws and/or sharp teeth? Or small and slithering, with a head full of murderous venom? You might find it surprising that the world’s deadliest animal — responsible for 750,000 deaths per year — has none of these formidable attributes. In fact, many people outside of certain geographical areas consider it to be nothing more than an itch-inducing, bombinating nuisance. That’s right — the mosquito reigns supreme as the world’s deadliest animal (sparing us from being our own worst enemy — humans directly cause the demise of 437,000 other humans per year, landing us second on the list of deadly animals).
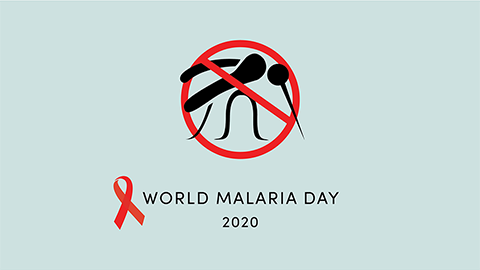
Devoid of any particularly savage biological features on its own, the mosquito has help in its claim to the crown: Its bite delivers a noxious payload in the form of tiny parasites or viruses. It is these miniature hitchhikers that go on to wreak havoc in the human body, often with deadly consequences.
Different species of mosquito — females only, as male mosquitos don’t bite (they adhere to a strictly vegan diet of plant sugars, and indulging in blood may even be harmful to them — play host to different infectious agents. Mosquitos of the genus Aedes transmit the “bone-breaking” viruses dengue and chikungunya — so called because of the debilitating joint and muscle pain they elicit — as well as Zika virus and the yellow fever virus. Culex mosquitos circulate West Nile virus. And yet a third genus, Anopheles, bears the Plasmodium parasites that cause malaria, making them the deadliest mosquito of them all: In 2018, malaria was responsible for 228 million infections and 405,000 deaths, 67% in children under 5 years of age.
In recognition of World Malaria Day, established in 2008 to draw attention to global efforts to control this deadly disease, let’s dig a little deeper into what malaria is and take a look at some exciting new advances published in ASBMB journals. (If you’re already well-versed in the basics, feel free to scroll on down to the article summaries.)
Clever organisms
Malaria is an ancient disease.
Its name comes from the Italian for “bad air,” as it was long believed to be a consequence of inhaling noxious substances lurking in the fetid air over swampy areas. It wasn’t until the 19th century that some started to suspect mosquitos were involved, and the true culprits — the parasites — weren’t properly inculpated until 1880, when a French surgeon named Alphonse Laveran caught sight of a wriggling parasite inside a patient’s red blood cell while working in an Algerian hospital.
Soon after, others identified and named four different species of parasite belonging to the genus Plasmodium, each responsible for a different “flavor” of malaria. (A fifth species has recently emerged as an important source of zoonotic infection.) The most common — and most deadly — of these is P. falciparum.
Although malaria cases occur in tropical and subtropical regions across the globe, Africa bears the brunt of the disease: A staggering 92% of cases occur there.
So what happens when a parasite-laden mosquito delivers a load of Plasmodium into a human victim?
Regardless of the infecting species, after an incubation period of seven to 30 days, a person stricken with malaria will typically experience some combination of fever, chills, sweats, headaches, nausea and vomiting, aches and malaise — and that’s just for what would be called an “uncomplicated” case. More severe consequences can include cerebral malaria (manifesting as neurologic abnormalities such as abnormal behavior, altered consciousness, seizures, and coma), severe anemia, acute respiratory distress syndrome, and acute kidney injury, among other medical emergencies. Young children and pregnant women are especially susceptible to severe symptoms.
But what exactly do these tiny organisms do inside the body that leads to such devastating effects? And how do they get into the mosquitos that transmit them? To answer these questions, let’s take a cursory look at the rather staggeringly complex lifecycle of Plasmodium parasites (with a figure from the CDC to help keep things straight).
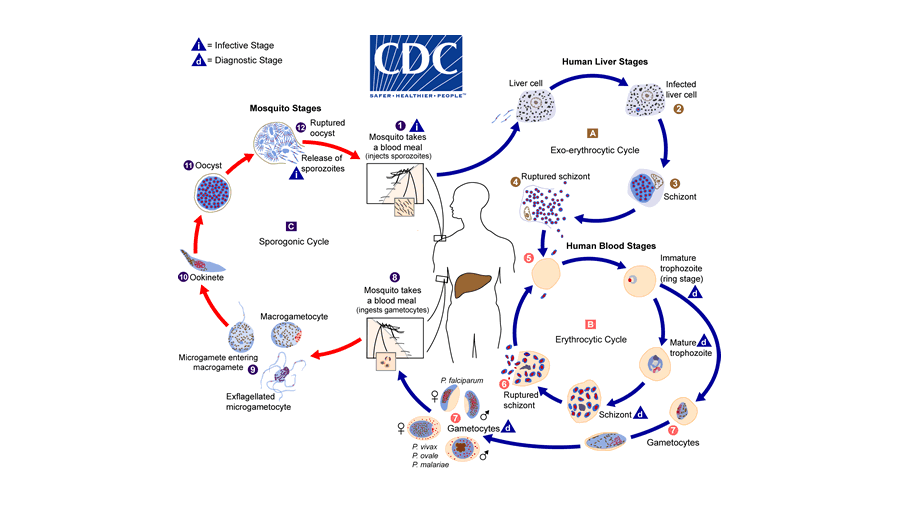
Over the course of evolution, these clever organisms have crafted a lifecycle that masterfully exploits for their own reproductive purposes the bodies of two very different hosts: mosquitos and humans.
When an infected mosquito takes a blood meal, she spits a payload of infectious parasites — called sporozoites — from her salivary glands into the skin of her human victim. Some of these sporozoites make their way to nearby blood vessels, which ferry them to the liver. Inside comfy and resource-replete liver cells, sporozoites mature into a new form called schizonts, each of which is bursting with six to 12 copies of yet another form, the merozoites. Next, the schizonts rupture, freeing the merozoites to launch the human blood stages of infection. After the merozoites invade red blood cells, they begin cycles of asexual reproduction, forming trophozoite ring forms that mature into schizonts before bursting their cellular incubators to release a new wave of infectious merozoites.
This cyclic bursting of red blood cells is the root of most common clinical manifestations: Numerous toxic substances from inside the RBCs flood the bloodstream, causing alarmed immune cells to ramp up production of various factors such as cytokines that mediate symptoms like fever and chills. In severe cases, the parasites demolish so many red blood cells that anemia results.
But these asexual merozoites alone are not sufficient to close the cycle of infection — if taken up by a mosquito as part of her blood meal, they are digested in her gut with no opportunity to infiltrate her salivary glands. To solve this problem and, as a bonus, also reap the evolutionary benefits of sexual reproduction, some parasites develop from the trophozoite ring form into not schizonts but sexual gametocytes. When these are devoured by an Anopheles mosquito, they not only survive her digestive tract but use her stomach as a breeding ground: Male and female gametocytes fuse into zygotes (or ookinetes), which nestle themselves into the wall of the mosquito’s midgut while they grow into oocysts. Similar to how schizonts encapsulate merozoites, oocysts contain multiple sporozoites that are released when the oocysts rupture. The sporozoites eventually migrate to the salivary glands of the mosquito, ready to hitch a ride into the next human their mosquito host takes a meal from.
What are our defenses against these tiny terrors? Evolution has provided natural protection, albeit in the form of a double-edged sword, to historically heavily affected populations: A range of hereditary blood disorders such as sickle cell anemia, the thalassemias, and G6PD deficiency provide a measure of resistance against the parasites, which are thwarted by the disordered red blood cells.
Repeated infections can also build some amount of immunity, particularly protective against severe symptoms.
For the rest of us, antimalarial drug regimens tailored to the patient’s clinical status, the infecting Plasmodium species, and drug-resistance statistics can provide effective treatment.
However, prevention by way of vector management has been the cornerstone of malaria control measures for many years — in endemic areas, insecticide-laced bed nets in particular have been instrumental in protecting people from bites while they sleep. In fact, incidence and mortality have been declining in recent years, but we still have a long way to go in our efforts to conquer these minuscule but formidable foes.
Research highlights
Current research efforts largely focus on forging a more detailed understanding of the complex malaria lifecycle, vaccine development (a daunting task given the plethora of different parasite forms and ability of the parasite to switch up its antigens), diagnostic improvement, drug development to combat resistance, and upgraded vector management tools (particularly as mosquito populations evolve insecticide resistance).
The following selection of articles recently published in ASBMB journals highlight specific advances in understanding host immunologic responses (important for vaccine development), making sense of basic parasite biology (key to developing new drugs), and illuminating the basis of drug resistance.
Deciphering host immunologic responses
Identification of one or more highly immunoprotective pathogen antigens is a required step for effective vaccine development. To widen the range of possible antigens that could form the basis of a malaria vaccine, Siau et al. screened sera from mice that were protected to different degrees from blood-stage infection with the murine malaria parasite P. yoelii against the entire parasite proteome. They then scored antigens to which the sera were reactive based on their predicted likelihood of mediating protection, providing an expanded list of protective antigens that could drive vaccine development. Read the full study in Molecular & Cellular Proteomics.
In another study published in MCP, Jaenisch et al. harnessed a powerful technology called high-density peptide arrays to provide highly granular insight into the specific epitopes on P. falciparum proteins that are recognized by the immune system. To make their peptide arrays, they laser-printed short segments of parasite proteins onto glass surfaces and then used them to screen sera from 27 malaria patients for reactivity. After correlating sera reactivity with immune status of each patient, the researchers were able to map immunogenic epitopes in the proteins, both confirming known immunogenic motifs and uncovering new ones that could constitute novel vaccine candidates.
Laying the groundwork not only for novel vaccine approaches but also for biomarker-based surveillance tools to predict patient vulnerability and potentially monitor immune effects of vaccines, Proietti et al. used machine learning to identify an immune signature that can accurately predict clinical protection against malaria. Using sera collected from a cohort in Ghana, the researchers correlated clinical immunity with IgG antibody responses to P. falciparum antigens to identify a core set of 15 antigens, IgG responses to which could be used to predict protection from infection with an accuracy of 86%. The 15-antigen signature was validated in a distinct cohort from Mali, strengthening evidence for its predictive value. Read more in MCP.
Elucidating basic parasite biology
The gametocyte stage, in which parasites differentiate into males and females, is crucial for parasite transmission. Miao et al. were interested in understanding differences in protein expression between male and female P. falciparum gametocytes (MG and FG). They separated the sexes and then analyzed their respective proteomes to identify a list of MG- and FG-specific proteins. The researchers noted a high degree of overlap, but also a substantial amount of divergence, between their results and sex-specific proteomes previously reported for P. berghei, a murine malaria species commonly used as a model in the lab. This study, published in MCP, provides a basis for a molecular understanding of sex-specific biology in P. falciparum.
To sustain replication in the relatively resource-poor environment of the red blood cells that it invades during human blood stages, P. falciparum must synthesize a number of essential nutrients and metabolites, including phospholipids (PLs) for membrane synthesis. Choline analogs — which antagonize the PL precursor choline — have been shown to be effective against malaria in clinical trials, raising interest in PL metabolism as a target for new antimalarials. In an article published in the Journal of Lipid Research, Wein et al. analyzed the contributions of different PL biosynthetic pathways present in P. falciparum to the synthesis of different types of PLs using deuterated precursor molecules and also determined the sources of these precursors. A clearer understanding of PL synthesis in P. falciparum could pave the way for development of drugs that target the pathways involved.
Plasmodium parasites translocate and invade host cells by means of a type of migration called gliding motility. Gliding motility is powered by a multiprotein complex called the glideosome, which is made of a core myosin motor that binds to actin in a manner similar to the system that enables skeletal muscle cell contraction. Although the glideosome is essential to Plasmodium pathogenesis, a molecular understanding of its components and how they interact with each other is lacking. In a study published in the Journal of Biological Chemistry, Bookwalter et al. report in vitro reconstitution of the glideosome core, which will enable identification of compounds that can inhibit its action.
Understanding drug resistance
Tetrahydrofolate is essential for the synthesis of several basic biological molecules, and higher eukaryotes — including humans — lack the enzymes to synthesize it; these attributes make the folate pathway an ideal target for anti-infectives, including the antimalarial sulfadoxine (SDX). SDX targets 6-hydroxymethyl-7,8-dihydropterin pyrophosphokinase-dihydropteroate synthase (HPPK–DHPS), a key player in tetrahydrofolate biosynthesis, but drug resistance mutations are spreading. In an article published in JBC, Yogavel et al. report the crystal structure of HPPK–DHPS and map five common drug-resistance mutations onto it to uncover the molecular basis of SDX resistance conferred by these mutations. This insight as well as other structural features of HPPK–DHPS could be used to develop new folate biosynthesis-targeting malaria drugs.
Some lighter reading
From JBC, an autobiographical account of a leading malaria researcher’s “improbable journey” into the world of malaria research (for more on her contributions to the field, also check out her profile in ASBMB Today as the 2018 recipient of the Alice and C.C. Wang Award in Molecular Parasitology, and from ASBMB Today, a slightly dated but still relevant look at P. vivax, the “other” malaria parasite.
Enjoy reading ASBMB Today?
Become a member to receive the print edition four times a year and the digital edition monthly.
Learn moreGet the latest from ASBMB Today
Enter your email address, and we’ll send you a weekly email with recent articles, interviews and more.
Latest in Science
Science highlights or most popular articles
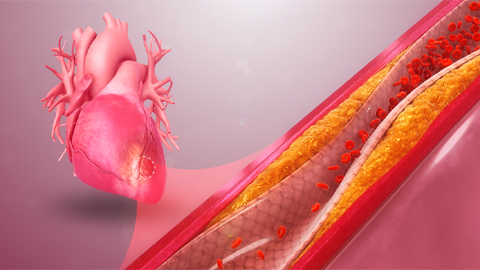
Scientists find unexpected correlation between age and HDL-C levels
In a 30-year multicenter study, researchers determined what factors predict HDL-C concentration. In their analysis, they found that HDL-C levels grew with increasing age and physical activity.
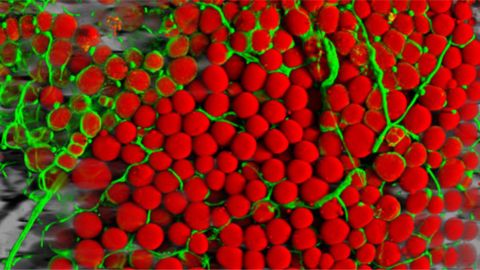
Butter, olive oil, coconut oil — what to choose?
Depending on the chain length and origin of the fat, regular fat consumption changes the specific makeup of fats in bloodstream and affect mild to severe cholesterol patterns. Read about this recent Journal of Lipid Research study.
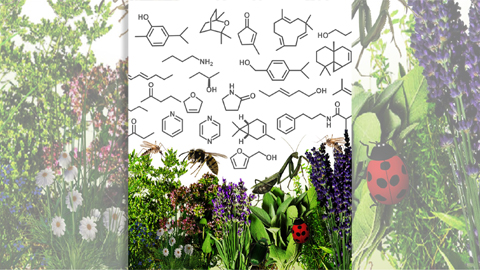
Computational tool helps scientists create novel bug sprays
Rapid discovery of mosquito repellent compounds is enabled through a novel screening platform that combines both computational modeling and functional screening.
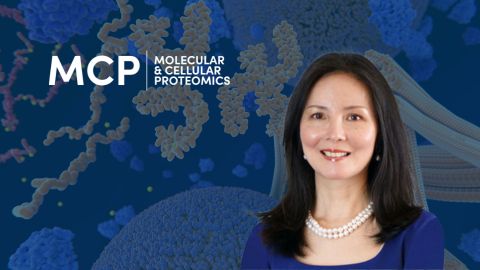
Meet Lan Huang
Molecular & Cellular Proteomics associate editor uses crosslinking mass spec to study protein–protein interactions to find novel therapeutics.
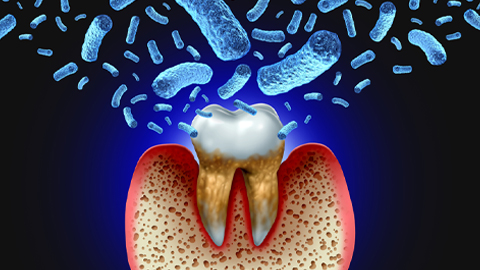
Influenza gets help from gum disease bacteria
Scientists discover that a protease from Porphyromonas gingivalis enhances viral spread. Read more about this recent Journal of Biological Chemistry paper.
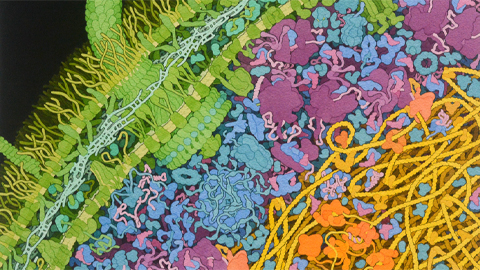
How bacteria fight back against promising antimicrobial peptide
Researchers find a mutation in E. coli that reduces its susceptibility to a potential novel antibiotic. Read more about this recent Journal of Biological Chemistry paper.