Sperm matters
There’s no delicate way to put this: Things can go wrong with the family jewels. Experts estimate that up to 50 percent of couples’ infertility cases stem from the men. But the problem, say the experts, is that the reasons for male infertility are largely unknown.
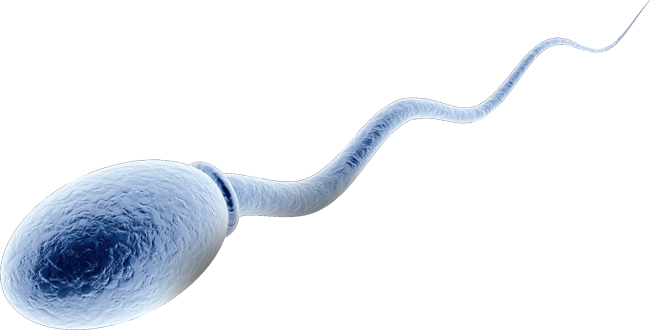
Although some genetic and lifestyle factors have been shown to affect sperm cells, “we have a very poor understanding of the basic mechanisms that regulate sperm production by the testes, the maturation and transit of the sperm through the male and female genital tracts, and events required for fertilization and early embryonic development,” says Dolores Lamb at the Baylor College of Medicine, the immediate past president of the American Society for Reproductive Medicine. “Because we don’t understand these molecular processes, we can’t diagnose” the causes of male infertility.
One would think that not understanding male infertility would drum up support for more research. After all, the future of the human race depends on it. But researchers say that is not the case. Male infertility research is largely in the hands of a small cohort of academic investigators backed by programs such as those at the U.S.’s Eunice Kennedy Shriver National Institute of Child Health and Human Development and REPROTRAIN, a program funded by the European Commission to prepare researchers to study male reproductive biology. In recent years, the pharmaceutical players in contraceptive and fertility treatments, such as Schering Plough, Organon and Wyeth, have withdrawn from reproductive biology research and development.
Experts say that much of the pharmaceutical industry’s lack of interest stems from the fact that in the past two decades assisted-reproduction clinics have been using a method that bypasses the need for functional sperm. Called intracytoplasmic sperm injection, or ICSI (pronounced ick-see), it now constitutes 68 percent of all assisted reproductive cycles done worldwide, according to ASRM. Because ICSI is effective in overcoming most forms of male infertility, Sheena Lewis at Queen’s University Belfast says, “There has been no impetus over the last 20 years to do any research on the basic molecular structures and functions of spermatozoa.”
Single-cell life
Because it is the only cell in the human body designed to leave the confines of the body, sperm genes echo those of unicellular life forms. An example is the sperm adenylate cyclase that produces cAMP. The sperm adenylate cyclase gene in homology studies bears the most resemblance to the adenylate cyclase found in cyanobacteria. “One way to think about the human genome is that it contains the memory of unicellular life. When the gametes are made, that genetic memory becomes expressed, and genes that are actually most similar to genes found in other unicellular organisms begin to be expressed in the testes,” says John Herr at the University of Virginia. “We call this the ancestral gene program that is activated” during sperm production. (Herr has developed two commercial home tests for male infertility, Sperm Check Fertility and Sperm Check Vasectomy, using biomarkers that are unique to the final stage of sperm development in the testes.)
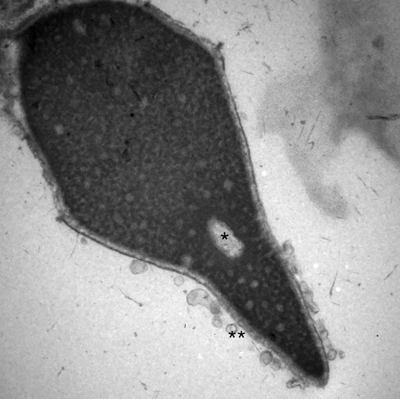
Sperm cells up close
So what do we know about sperm cells? There are certainly a lot of them. A fertile male usually churns out between 5 million to 250 million sperm cells per milliliter of ejaculate (the average human ejaculate is between 0.75 mL and 2.5 mL). Out of the millions, fewer than a hundred sperm cells actually arrive at the oocyte. And out of those, only one can fertilize the oocyte. It’s the sperm version of the TV show “Survivor.”
Human sperm are produced in several steps. Testicular stem cells undergo mitotic and then meiotic cell division over the course of about 70 days. The result is spermatids, which then proceed onto terminal differentiation. These round haploid cells undergo a series of dramatic morphological changes into the long, polarized sperm cells known also as spermatozoa.
In an ideal human sperm, there is a smooth, oval-shaped 5-micrometer head free of indentations, bulges or tapers. In it sits a sacklike organelle, called the acrosome, with various lytic enzymes that help to break down the glycoprotein shell around the oocyte. The paternal genetic material of 23 chromosomes is crammed in the nucleus next to the acrosome. About 90 percent of the genetic material is condensed by small, arginine-rich proteins called protamines; the remaining 10 percent is wrapped around histones. Next to the head is the midpiece, which houses mitochondria. And then there’s the tail, which propels the sperm to the egg. The work of the tail is done by a complex microtubule structure known as the flagellum or axoneme. At the axoneme’s base are the centrioles. A structure called the fibrous sheath is wrapped around the axoneme.
Sperm cells are carried out of the man in the semen, which is produced in the seminal vesicles, prostate gland and urethral glands. Once the sperm are inside the female genital tract, they undergo a subsequent maturation process called capacitation that gears them up for fertilization. (See sidebar.)
Molecular mysteries
From biochemical and molecular biology standpoints, sperm cells are puzzling. As spermatids go on to become spermatozoa, “they shed a lot of cytoplasm and the normal molecular biology machinery,” says Joseph Tash at the Kansas University Medical Center. This is in stark contrast with what happens to somatic cells, which are filled with the cytoplasm, a fluid that provides signaling molecules a medium through which to move. Indeed, several experts refer to the sperm interior as being a solid state. Charles Muller, director of the Male Fertility Laboratory at the University of Washington, explains, “In sperm, the membranes of one organelle are pretty much placed up against the membranes of another.”
The sperm cell uses second messengers, such as cyclic AMP and GMP, which are soluble messengers in the somatic-cell book. “Do those second messengers function in the same way as a soluble second messenger in a sperm cell that has virtually no cytoplasm? Or do they function as second messengers more in a solid-state environment? Those are really interesting questions to ask,” says Gregory S. Kopf, also at KUMC and the former director of the preclinical male contraceptive research and development program at Wyeth, which is now part of Pfizer.
That conventional signaling molecules may work differently in sperm cells is indicated during capacitation, when some proteins undergo tyrosine phosphorylation. If these tyrosine phosphorylation events do not occur, a sperm cell cannot complete capacitation and fertilize an egg. “The conundrum is that cAMP is important for these changes, but cAMP in every other cell type does not directly trigger tyrosine phosphorylation. cAMP triggers threonine and serine amino acid residues to be phosphorylated,” says Tash. “The question of how cAMP triggers tyrosine phosphorylations in sperm is still unanswered.”
The highly segmented character of the sperm cells raises other questions. How does biochemical information get from one compartment to another? “Many people have argued over the years that there’s actually no clear path for cytoplasmic molecules to move from the head into the midpiece or tail. There do seem to be constrictions and boundaries there that might form a structural barrier to any interchange,” says Muller. A case in point is ATP.
To do all that swimming and fertilizing, sperm cells need a lot of energy. How exactly does a sperm cell meet its energy requirements? The mitochondria in the sperm midpiece churn out ATP by oxidative phosphorylation. “How does that ATP get down to the dynein ATPases that are along the axoneme in the middle of the tail? That’s a long distance,” says Muller.
It turns out that the ATP in the tail comes from glycolysis, a topic in which Deborah O’Brien at the University of North Carolina in Chapel Hill is an expert. “We learn about glycolysis in college as this kind of boring, immutable metabolic pathway, right?” she says. “Well, sperm cells have modified nearly every step of that pathway.”
Several glycolytic enzymes are distinct in sperm and may illustrate how sperm cells have adapted a metabolic pathway to occur essentially in the solid state. Multiple glycolytic enzymes are pinned to the fibrous sheath so firmly that O’Brien says attempts to strip them off the sheath, even with 6 M urea, potassium thiocyanate and detergents, fail. If any of these three glycolytic genes are knocked out in mice, the males are infertile. Thus, this sperm-specific glycolytic pathway is “a pathway that’s essential for sperm function,” sums up O’Brien.
Besides oxidative phosphorylation and glycolysis, which derive ATP from sugars, sperm also can get their ATP from fatty acids metabolized in mitochondrial and peroxisomal pathways. In a paper published in Molecular & Cellular Proteomics earlier this year, a team led by Rafael Oliva, REPROTRAIN’s project coordinator, and Alexandra Amaral at the University of Barcelona discovered a number of peroxisomal proteins in the tails of healthy human sperm. This came as a surprise, because the conventional wisdom was that sperm didn’t have peroxisomes. Some peroxisomal proteins are involved in the oxidation of very long-chain fatty acids. The implications, Amaral says, are that “sperm might be able to use fatty acids as fuel, and lipidic beta oxidation may contribute to sperm motility.”
Even if the sperm make it to the oocyte, the state of the sperm DNA can greatly influence male fertility. Experts say the tightly wrapped DNA in the sperm head gives the impression that the genetic material is protected. But that’s not the case. Because much of the molecular machinery is taken out of the cell toward the end of making terminally differentiated sperm, the final cells don’t have the tools for repairing damaged DNA. With the mitochondria in the midpiece, the DNA sits right next to organelles that spew out reactive oxygen species that damage DNA. With the loss of transcriptional machinery during differentiation, sperm have lost the primary surveillance system for identifying ROS-induced damage. ROS aren’t the only things to damage DNA. Environmental toxins, lifestyle choices such as smoking, and stresses all damage DNA.
Even with damaged DNA, sperm can fertilize eggs. The oocyte’s DNA repair machinery can fix most single-strand breaks in the paternal DNA. However, researchers have observed that there are a notable number of double-strand breaks in the sperm DNA. “There is no way those can be put back in the right places,” says Muller. “We don’t know of a mechanism of DNA repair that can handle that. There is no template.” This has implications for older men, because age has been suggested to be a factor in creating more paternal DNA damage. For older men attempting to conceive babies, the highly damaged paternal DNA could stop fertilization from happening or, even if fertilization does happen, cause problems with fetal development.
For a long while, conventional wisdom held that sperm cells didn’t have any RNA because the DNA was transcriptionally silent. In recent years, work by David Miller at the University of Leeds in the U.K. and others demonstrated that there are messenger and noncoding RNAs in sperm. But their role is unclear. Miller says that there are hints that the sperm RNA is responsible for embryonic gene activation. He also speculates that the RNA acts as a compatibility signal to the egg, telling it that the invading sperm cell is not a pathogen.
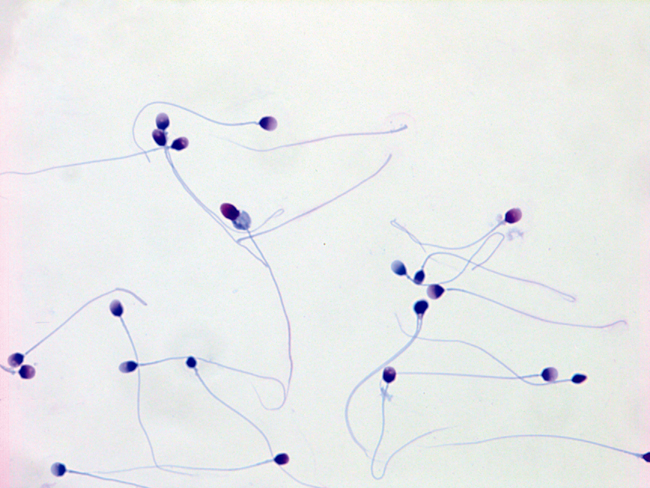
ICSI
The first demonstration of assisted reproduction was in vitro fertilization and the birth of Louise Brown in 1978. Robert Edwards, the emeritus professor at the University of Cambridge who died last month, won the Nobel Prize in 2010 for developing IVF, a process in which sperm cells and eggs are incubated in cell culture dishes for fertilization to take place.
But IVF can’t tackle all fertility problems. If a man’s sperm cells are few in number, have poor motility or carry morphological defects, they are not going to fertilize an oocyte even in a cell-culture dish. Until ICSI came along in the1990s, “there used to be no hope for these men,” says Muller.
The technique, which bypassed the need for culture-dish fertilization, was developed by Gianpiero Palermo, currently at the Weill Cornell Medical College, while on sabbatical in Belgium. The first ICSI baby was born in January 1992 in Belgium. Approximately 4 million have been born by IVF in the past 35 years; 2 million babies have been born worldwide by ICSI in the past two decades.
For ICSI, a technician scans a semen sample under an optical microscope and picks out a single sperm cell that appears to be normal. Then the technician moves that sperm cell with micromanipulators and directly injects it into the cytoplasm of a waiting egg.
ICSI works not only with terminally differentiated sperm cells but also with immature sperm cells plucked from a man’s testes. Lewis says because ICSI allows technicians to use sperm that normally wouldn’t be able to fertilize oocytes on their own, it provides a way “to bypass all the laws of nature.”
Experts say the method’s success has killed the pharmaceutical industry’s interest in understanding the fundamental biology of sperm and developing male infertility treatments. “They think ICSI is the great panacea,” says Miller. “There are two arguments which factor against those of us who are working in the male reproductive field. Because of the advent of ICSI, they think that it has solved the problem of male infertility. The second thing is that even if we do discover what causes male infertility, they think there is nothing we can do about it. There is no translational benefit … They would turn around and say, ‘There’s nothing you can do about it. Just do ICSI and be done with it.’”
But Miller and others say that those arguments are fallacious because there may be other factors that lead to infertility that ICSI can’t help. ICSI is based on sperm that seem morphologically fine under a microscope. “People have always made the assumption that the really nice looking sperm must be the best,” says Muller. “That’s a completely unsupported assumption.”
Muller describes a couple he encountered who had visited a clinic that “believed ICSI was the answer to everything,” he says. “They got zero fertilization. They spent $10,000 and got nothing out of it.”
After the failed attempt, the couple went to Muller, who recounts seeing under the microscope that the man’s sperm cells were devoid of acrosomes. One component in or near the acrosomes is phospholipase Cζ, which is postulated to be an activating factor that helps the fertilized oocyte initiate the first cell division. The enzyme triggers a calcium signaling cascade, so Muller’s group attempted to trigger the calcium cascade with an ionophore. “Lo and behold, they got five out of six embryos developing, and the couple is currently pregnant!” Muller says.
This example and others like it point to the fact that sperm cells are not mere donors of paternal genes. Besides phospholipase Cζ, sperm also must donate their centrioles to the fertilized egg, a discovery made by Palermo and colleagues in 1994 using ICSI. Miller’s work on RNA points to the possibility that sperm cells that don’t contain the right RNA molecules may be ineffective for fertilization. There is probably a host of other molecules that sperm contributes to the fertilization process that haven’t yet been identified.
Supporting reproductive biology
Given the heartache of infertility and the cost of treatments, experts argue that sperm biology needs to be investigated more thoroughly. One in five couples requires reproductive assistance, and a single round of IVF can cost up to $17,000. Data from the European Human Society of Reproduction and Embryology show that IVF and other assisted-reproduction technologies have had the same success rate over the past 30 years, somewhere between 25 and 30 percent. “We haven’t really made a lot of progress,” says Lewis. “A lot of things have been done to tweak superovulation regimens and things like that. But little has been done about the sperm.”
Then there is the health aspect. Men who resort to ICSI usually don’t get an actual diagnosis to understand why they are not producing healthy sperm in the first place, say Lamb and Oliva. Often, the lack of sperm can point to a more general health problem. For example, men with DNA-repair problems don’t produce functional sperm, and there are associations between poor sperm counts and cancer in later life. Oliva also points out that, because the men are not diagnosed, if there are any genetic issues with their sperm, ICSI just passes those issues to the next generation.
So the current situation with male infertility is worrying, say experts who are working hard to understand the molecular and biochemical basis for sperm malfunction. “We cannot be treating infertility the way we are treating it now, by just taking one single sperm cell and injecting into an oocyte,” says Oliva. “It seems to solve the problem, but it’s temporary. The next generation is going to have the same problems as we have now.”
Enjoy reading ASBMB Today?
Become a member to receive the print edition four times a year and the digital edition monthly.
Learn moreGet the latest from ASBMB Today
Enter your email address, and we’ll send you a weekly email with recent articles, interviews and more.
Latest in Science
Science highlights or most popular articles
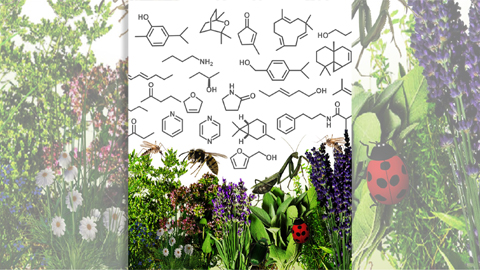
Computational tool helps scientists create novel bug sprays
Rapid discovery of mosquito repellent compounds is enabled through a novel screening platform that combines both computational modeling and functional screening.
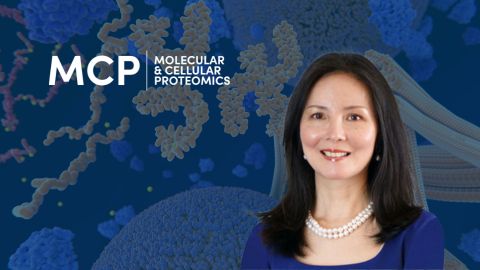
Meet Lan Huang
Molecular & Cellular Proteomics associate editor uses crosslinking mass spec to study protein–protein interactions to find novel therapeutics.
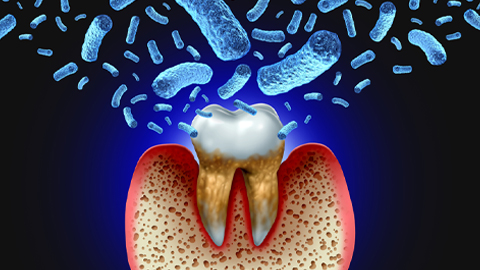
Influenza gets help from gum disease bacteria
Scientists discover that a protease from Porphyromonas gingivalis enhances viral spread. Read more about this recent Journal of Biological Chemistry paper.
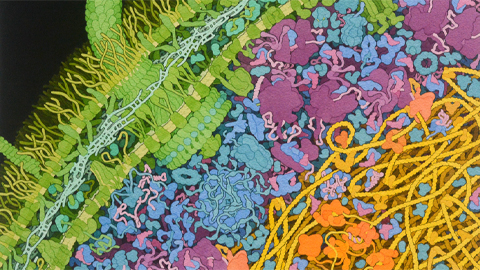
How bacteria fight back against promising antimicrobial peptide
Researchers find a mutation in E. coli that reduces its susceptibility to a potential novel antibiotic. Read more about this recent Journal of Biological Chemistry paper.
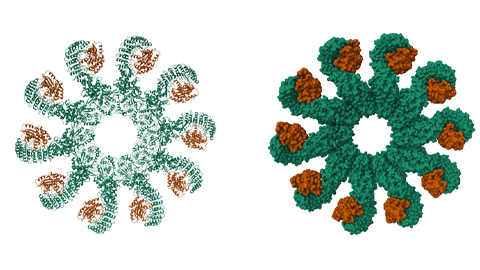
New clues reveal how cells respond to stress
Redox signaling protein may help regulate inflammasome and innate immune activation. Read more about this recent Journal of Biological Chemistry paper.
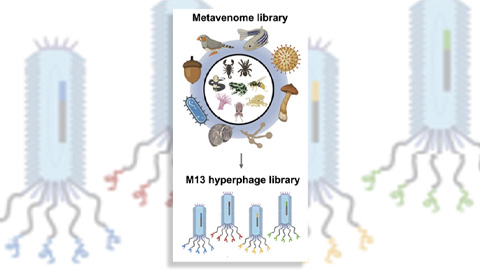
Innovative platform empowers scientists to transform venoms into therapeutics
Scientists combine phage display and a “metavenome” library to discover new drugs that bind clinically relevant human cell receptors. Read about this recent Molecular & Cellular Proteomics paper.