The birth of genetics
In 1854, a monk with remarkable intellectual curiosity by the name of Gregor Mendel began to grow peas in the greenhouse of the St. Thomas Monastery in what is now the Czech Republic. By 1865, Mendel had made a series of observations that ultimately changed the fundamental understanding of how traits are inherited by living organisms. He presented his key findings about what he termed “certain laws of inheritance” in 1865, and subsequently his studies were largely ignored by the scientific community for the next 35 years. Why?
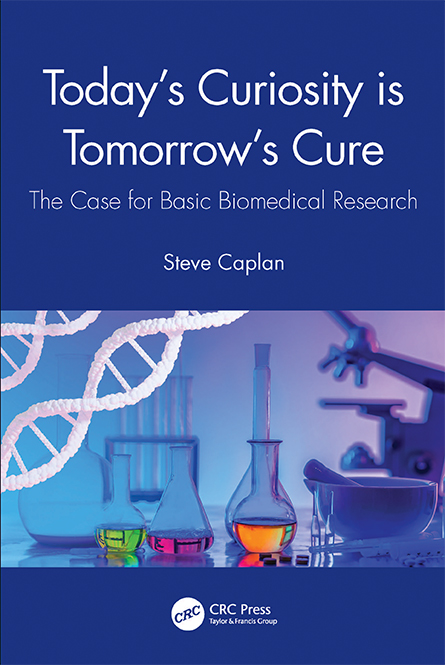
Consistent with the idea that the timing of scientific discoveries needs to be appropriate for those findings to be properly appreciated, Mendel’s laws of inheritance preceded the large-scale acceptance of Darwin’s theory of evolution. Indeed, it was not until the early1900s that widespread knowledge of Mendel’s laws was propagated, and it took even longer until his ideas were fully accepted.
At the turn of the 20th century, three new papers were published, each of which rediscovered Mendel’s laws of inheritance. In the prolog of her 2000 book “The Monk in the Garden,” Robin Marantz Henig noted, “The explanation usually given for this curious turn of events is that the world wasn’t ready for Mendel’s laws in 1865, and that by 1900, it was.”
Whether Mendel was a genius or simply a seasoned plant breeder who happened to be in the right place at the right time — an issue debated by some scholars and historians — is, for the most part, irrelevant. What is significant is that he was clearly among the first researchers to observe and publish findings that showed that traits are inherited, and he outlined predictable and strict mathematical rules that govern the passing of individual traits from parent to offspring as discrete particulate units that exist in pairs in all individuals.
A raging battle
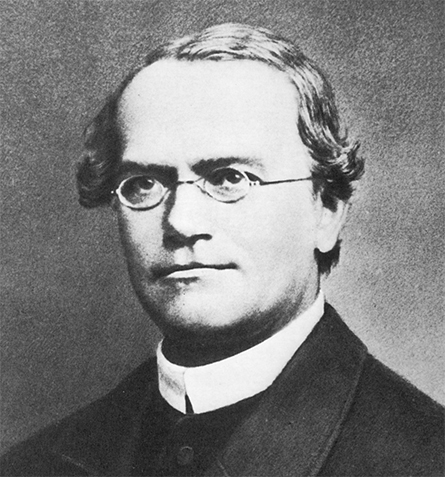
a mathematician and an Augustinian friar.
Perhaps one of the main reasons why Mendel’s work remained relatively obscure until the early 1900s was that the entity known as a gene remained so nebulous in the absence of a molecular understanding of what that entity entailed. The lack of a firm understanding of how genetic material is passed from generation to generation — or more accurately, what comprises that genetic material — made it extremely difficult for contemporary scientists to accept Mendelian genetics.
Indeed, a great debate erupted following the publication of Cambridge researcher William Bateson’s 1894 book “Materials for the Study of Variation: Treated with Especial Regard to Discontinuity in the Origin of Species,” in which he outlined 886 examples of discontinuous variation in heredity. Bateson, who, upon reading Mendel’s studies on the genetics of peas years earlier, reportedly felt that he had been scooped by Mendel, became one of the biggest advocates of Mendelian genetics. The idea that genetic traits could skip generations as a result of being recessive — meaning that the trait is only passed on to offspring if it is inherited from both parents — was somewhat revolutionary in that it seemingly opposed some of the new ideas that had been emerging from modern statistics.
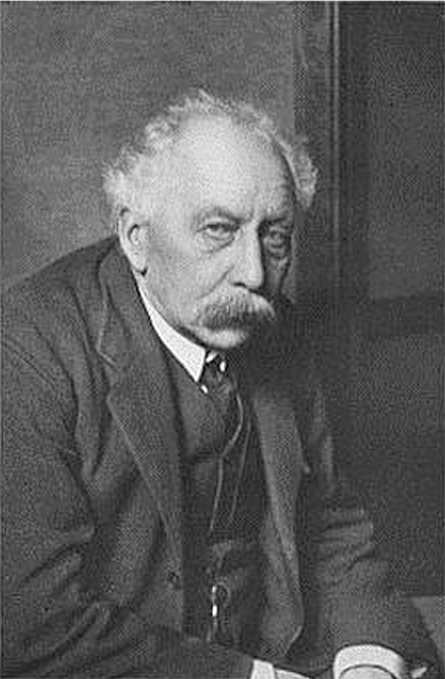
Around the time of Mendel, in the late 1800s, Francis Galton discovered the statistical concept of regression to the mean; simply put,if a sample point is extreme when observing random variables, then additional points observed in the future will more likely be closer to the mean and are less likely to be outliers. Galton calculated that Darwin’s evolution must occur by larger, discontinuous steps rather than by small, incremental ones to prevent regression back to the mean.
The scientists who favored the notion that evolution was a smooth and continuous occurrence were known as biometricians. Bateson and those who supported the Mendelian model were convinced that only discontinuity could explain inheritance of many traits, and thus a raging battle was fought in a series of letters and counterletters published in the journal Nature.
A chromosome theory
By the early 1900s, however, more evidence in support of Mendel’s ideas was coming from a different direction. In particular, two scientists, Walter Sutton and Theodor Boveri, contributed greatly to this enterprise.
Sutton did significant research under the tutelage of the famous Edmund B. Wilson at Columbia University in New York, publishing “The Chromosomes in Heredity” in 1903 with the conclusion that chromosomes (which were now visible under the microscope by new cytological techniques) carry Mendel’s hereditary material.
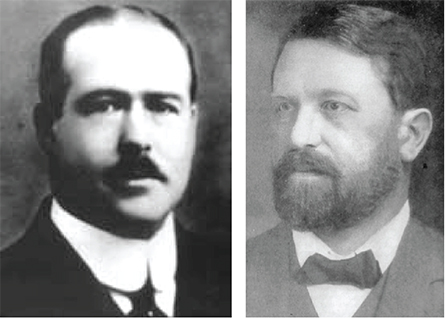
that Mendelian laws of inheritance could be applied to chromosomes.
The German cytologist, cell biologist and zoologist Boveri had a remarkable career during which he made great discoveries, often relying on his zoological experience to make use of interesting systems to study. For example, he took advantage of fertilized sea urchin eggs and later the nematode Ascaris megalocephala, a parasite of the horse gut that later in his life infected him and may have caused his death. Boveri studied the centrosome or what he termed the “centrosoma” and documented its significance for cell division. He also found that the centrosome itself divides and organizes the surrounding cytoplasm in such a manner that the spindle fibers radiate from it and contact the chromosomes. Presciently, Boveri also published a lesser known study in which he proposed that aberrant chromosomes might even be responsible for the generation of cancers in his 1914 book “Concerning the Origin of Malignant Tumors.”
The work of these two scientists advanced the idea of Mendelian genetics greatly and led to what is known as the Boveri–Sutton chromosome theory.
A hereditary twist
Further support for Mendelian genetics came from the work of Nettie Stevens, who had to be an extraordinarily brilliant scientist to overcome the rampant misogyny of her era. Stevens was a geneticist who trained in the laboratory of Thomas Hunt Morgan, a famous fly geneticist and Nobel laureate for his contributions to chromosomes and genetics at Bryn Mawr, where Edmund Wilson was also a faculty member. In the course of her doctoral studies, Stevens received a fellowship to travel to Germany and train with Boveri before completing her Ph.D.
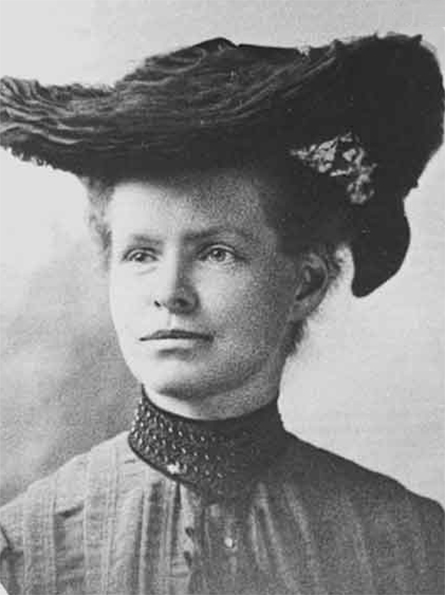
Upon returning to the U.S., Stevens worked on mealworms (Tenebrio molitor) and observed that somatic cells of female mealworms contained 20 large chromosomes, whereas those of the male mealworm had 19 large chromosomes and one small one. She also found that exactly half the spermatozoa cells from the males contained nine large chromosomes and one small chromosome, whereas the other half had 10 large chromosomes. Her conclusion was that the eggs fertilized by the sperm with the 10 large chromosomes gave rise to female mealworms, and therefore the small chromosome dictated the generation of male mealworms. Her discoveries were further validated by Wilson when he looked at chromosome numbers in numerous species of the insect Hemiptera, thus supporting Mendelian genetics and providing a new twist to the mechanisms of heredity.
With support from the findings of great cytologists and cell biologists, Mendelian genetics won the day. However, Mendelian genetics were ultimately combined with Galton’s mathematical advances to yield new statistical methods and give birth to the modern field of genetics. All that was missing was an understanding of what constituted the particulate hereditary element within cells and chromosomes that allowed for the passage of traits from one generation to the next. Thus, the field of genetics was born through observations and careful experimentation, but without understanding the role of DNA in this process, scientists were still looking at the tip of the iceberg.
This article is an excerpt from Steve Caplan’s new book, “Today’s Curiosity Is Tomorrow’s Cure: The Case for Basic Biomedical Research,” published by CRC Press. It has been edited for ASBMB Today.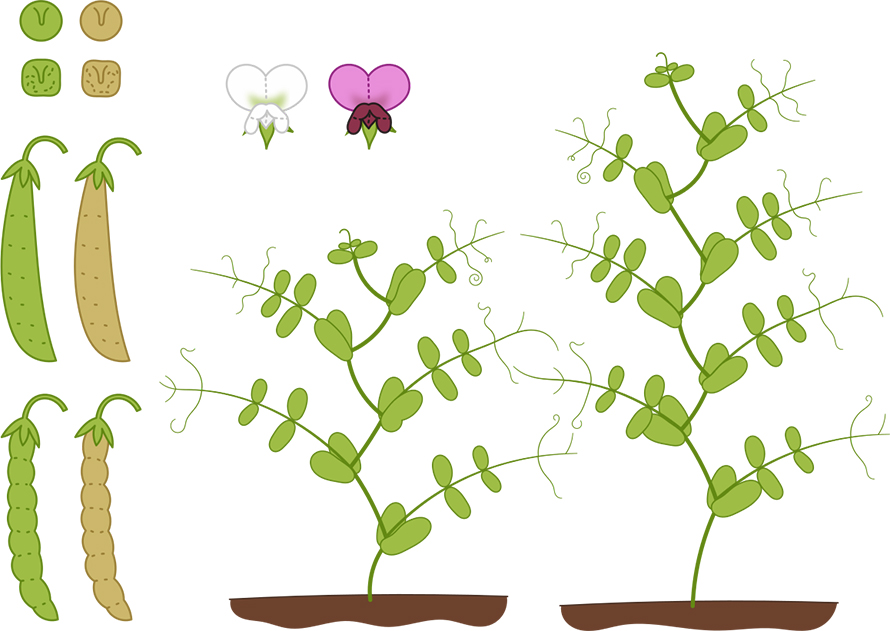
Enjoy reading ASBMB Today?
Become a member to receive the print edition four times a year and the digital edition weekly.
Learn moreGet the latest from ASBMB Today
Enter your email address, and we’ll send you a weekly email with recent articles, interviews and more.
Latest in Science
Science highlights or most popular articles
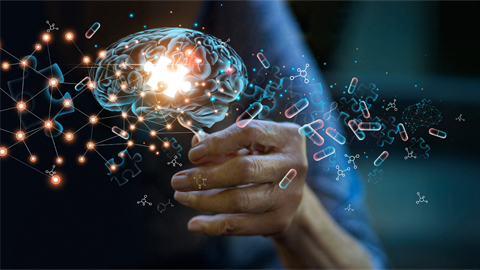
Does a protein hold the key to Alzheimer’s?
Researchers in Maryland and Massachusetts team up to study how SORL1 promotes tau trafficking and seeding in cells that leads to the neurodegenerative disorder.
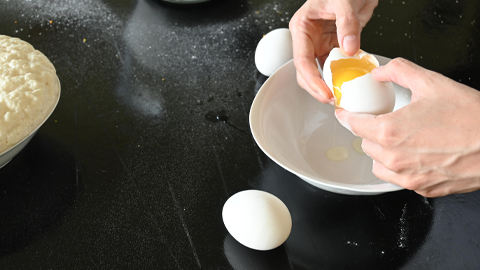
Cracking the recipe for perfect plant-based eggs
It involves finding just the right proteins. With new ingredients and processes, the next generation of substitutes will be not just more egg-like, but potentially more nutritious.
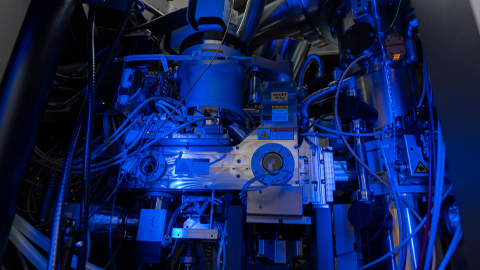
MSU researchers leverage cryo-EM for decades-in-the-making breakthrough
Lee Kroos and Ben Orlando have reported the first high-resolution experimentally determined structures of the intramembrane protease SpolVFB.
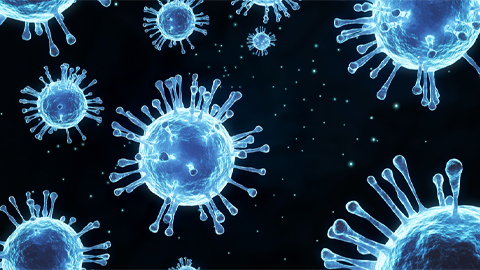
From the Journals: MCP
Rapid and precise SARS-CoV-2 detection using mass spec. Mapping brain changes from drug addiction. Decoding plant osmotic stress response. Read about recent MCP papers on these topics.
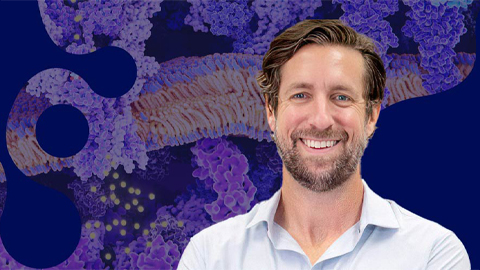
What seems dead may not be dead
Vincent Tagliabracci will receive the Earl and Thressa Stadtman Distinguished Scientist Award at the ASBMB Annual Meeting, April 12–15 in Chicago.
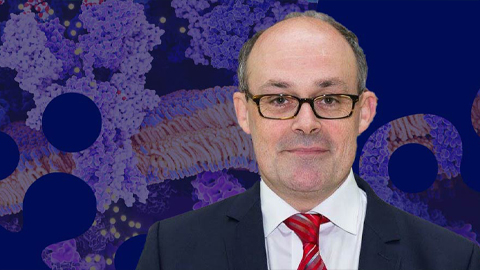
'You can't afford to be 15 years behind the parasite'
David Fidock will receive the Alice and C.C. Wang Award in Molecular Parasitology at the 2025 ASBMB Annual Meeting, April 12–15 in Chicago.