A cold day in Stockholm
Camillo Golgi couldn’t stop thinking about Santiago Ramón y Cajal.
As the wan December sun began to rise on icy Stockholm, his mind turned again to his extroverted Spanish antagonist. Thirty-three years earlier, in 1873, Golgi had developed the black reaction, a technique that revealed for the first time the elegant detail of a neuron in its entirety. His work had launched one of the most profound scientific explorations of the era — the study of the brain at the cellular level — and established Golgi as a pioneer in the field.
The Nobel Prize committee had beckoned Golgi to the Swedish capital to be feted for his accomplishments. Under different circumstances the recognition might have been a gratifying moment of self-reflection for the reserved and ever-busy Italian. But for Golgi, it would be the last stand in an increasingly bitter fight.
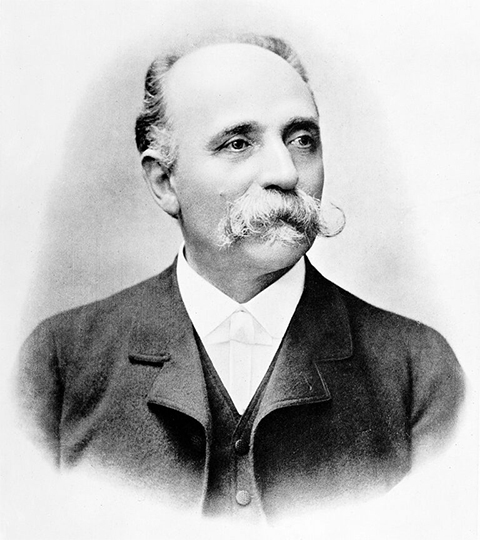
In the three long decades since Golgi first devised the technique that he said could “demonstrate, even to the blind,” the structure of the brain’s tissue, his misguided devotion to an outdated theory had chipped away at his reputation. Once mainstream, his concept of the nervous system was now a relic. In the intervening years, his rival, Cajal, had modified and refined Golgi’s black reaction to advance a new paradigm, an effort Cajal had declared, in his boisterous manner, “an act of rebellion” against the status quo — and, by extension, Golgi.
By 1906 Cajal had supplanted the Italian, discovering through his microscope elusive truths that had shaped the modern study of the nervous system. He, too, was in Stockholm that December. The prize was to be shared.
As Swedish anatomist and Nobel committee member Gustaf Retzius said, Golgi supplied “the key to open the building that encloses the secrets of the nervous system, but only Ramón y Cajal taught us how to use it.”
Frost clung to the coats of scholars and scientists as they strolled through the doors of the Royal Academy of Music just before noon on Dec. 11, entering a hall ornately decorated for the occasion. An enormous laurel wreath covered in blue and gold ribbons loomed behind the stage, where the Italian and Spanish flags were displayed together, alongside a bust of Alfred Nobel himself. The guests were all there to recognize the inextricable achievements of these two rivals who had only just met for the first time.
Golgi’s speech that day would be the culmination of a dispute in the nascent days of neuroscience that was driven by ego and interpretation — a struggle to establish the theoretical foundation from which investigators would venture into the unknown depths of the brain. As he prepared to address the international scientific community, Golgi refused to yield.
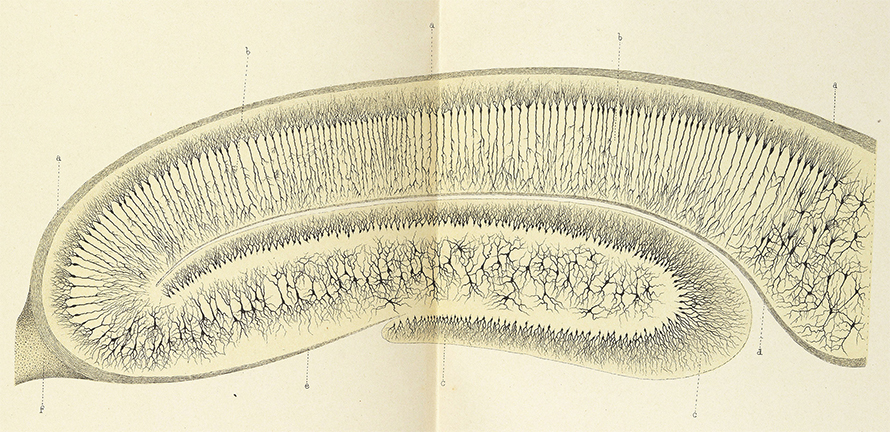
The sacred fire of scientific work
Camillo Golgi was hardly destined for Stockholm. Born in 1843 in a humble house in Corteno, a tiny village in the Italian Alps, he was “quiet, thoughtful, methodical and patient,” in the eyes of his father, a physician whose path Golgi followed to the University of Pavia’s medical school.
At the time, Italy’s universities were slowly emerging from a period of decline. Young Italian academics trained in the more illustrious scientific communities of Germany and Austria were returning home to spread the gospel of experimental medicine.
With Italy’s unification process, il Risorgimento, inspiring a wave of ideological change, the intensely patriotic Golgi was swept up in the positivist movement that enshrined science and the experimental method — “a weapon forged by the new school,” as he called it — as the path toward unending progress. He was driven by scientific curiosity, particularly of the central nervous system, and committed himself to researching its function with exceptional rigor. But the experimental method is only as effective as the malleability of the experimenter’s mind, and Golgi’s rigid nature would eventually stand in his way.
For Golgi, the pursuit of scientific knowledge was “a battle not of grandiose ideas but of facts accumulated with the pertinacity of patient and tireless work” that would open the way to “new conquests.” Although fiercely determined internally, he was placid on the surface, a shy man of few words who eagerly gave his spare time to research. Long after he died, his niece, Carolina, whom Golgi and his wife, Lina, had adopted in lieu of children of their own, remarked that he “lived with his thoughts” and could scarcely be described beyond his life as a scholar.
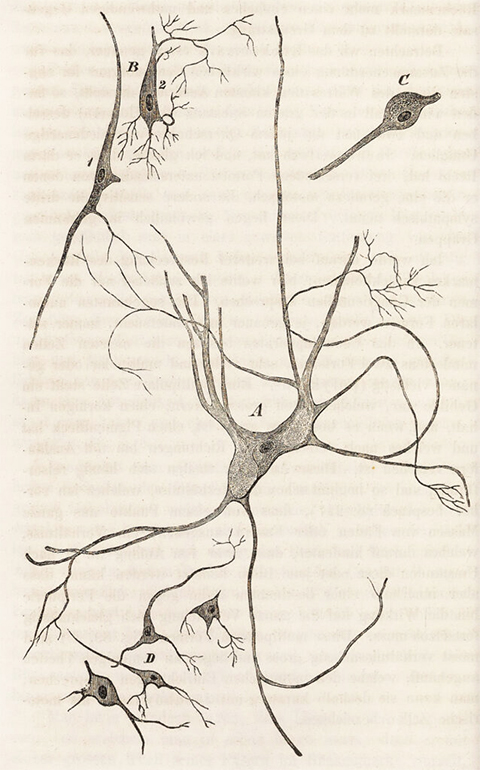
By the late 1860s, he was conducting his first histological studies of nerves while working at a clinic for patients with mental disorders. In the experimental pathology laboratory of Pavian professor Giulio Bizzozero, who later identified the role of platelets in coagulation, Golgi had access for the first time to microscopes and tools for vivisection. He took as his bible Rudolf Virchow’s "Cellular Pathology," an influential tome that declared each cell in an organism originates from another cell and identified cellular dysfunction as the cause of disease. Golgi began experimenting with a variety of staining substances and fixatives, learning how to most effectively prepare nervous tissue for examination.
For an emerging histologist, staining was the gateway to a new world. The right combination of preservatives and dyes could transform a colorless, transparent piece of tissue into a legible microscopic landscape whose cellular structures could be discerned among an otherwise muddled mass. But the science was still developing, and researchers sought better methods to see more clearly what lay before them.
In 1872 Golgi was appointed chief physician of a hospital for the terminally ill in Abbiategrasso, a town on the western outskirts of Milan, where he could study tissue samples collected from twice-monthly postmortem exams. His responsibilities were minimal, allowing seemingly endless time to indulge his scientific curiosity. He yearned “to penetrate the secrets of the nervous tissue, the most noble and mysterious tissue,” a student of his later wrote. “Trained to work with limited means and rich with the sacred fire of scientific work,” as he later said, he set up a rudimentary lab in the kitchen of his modest apartment, where he toiled by candlelight at the microscope. He devoted himself to his “coveted goal” with “religious fervor” and “single-minded perseverance,” a friend and colleague wrote. Within a year, he had found the novel staining technique that would break open the field of neuroscience.
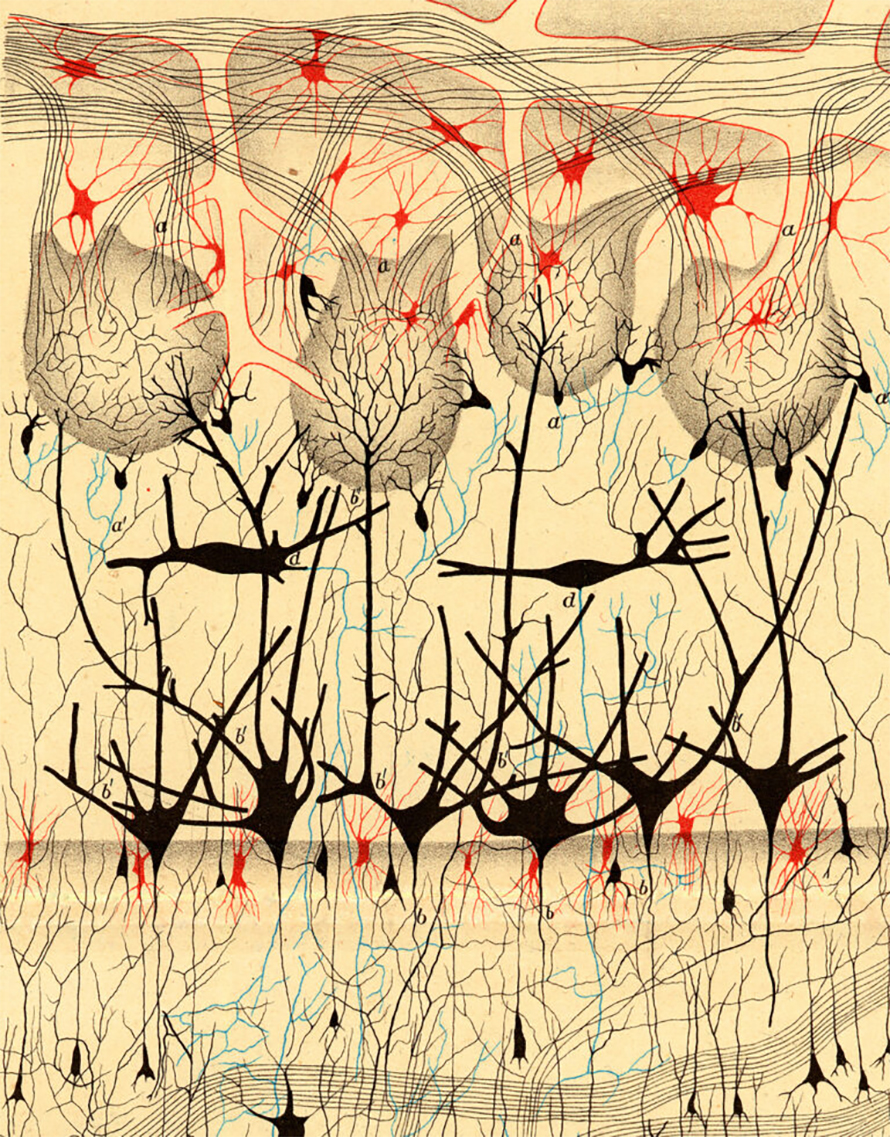
Contrast and control
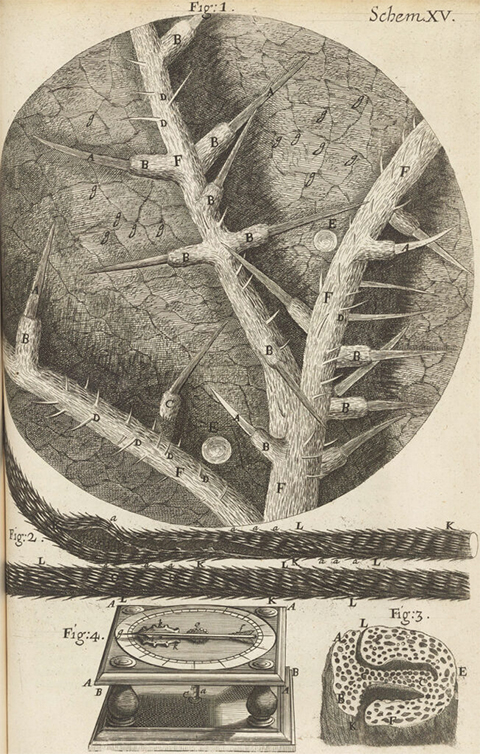
Shortly after Galileo used the telescope to peer into the infinite, English polymath Robert Hooke applied the microscope to the infinitesimal. In his landmark "Micrographia" (1665), Hooke used a homemade compound microscope to describe and illustrate the minute structure of insects, plants and objects, including dyed hair and wool. The work became the first blockbuster science book and coined the term "cell," but it took more than 50 years for another scientist to deliberately stain a biological object for research.
Inspired by "Micrographia," Dutch scientist Antonie van Leeuwenhoek spent his life building hundreds of microscopes, mastering lens-grinding and flamework techniques that allowed him to look closely at anything that caught his interest, including the “wee animalcules” — single-celled organisms, such as bacteria and protozoa — that he discovered. His use of saffron to help visualize the microscopic structure of transparent tissue in 1719 presaged the histological techniques Golgi and Cajal established so many years later.
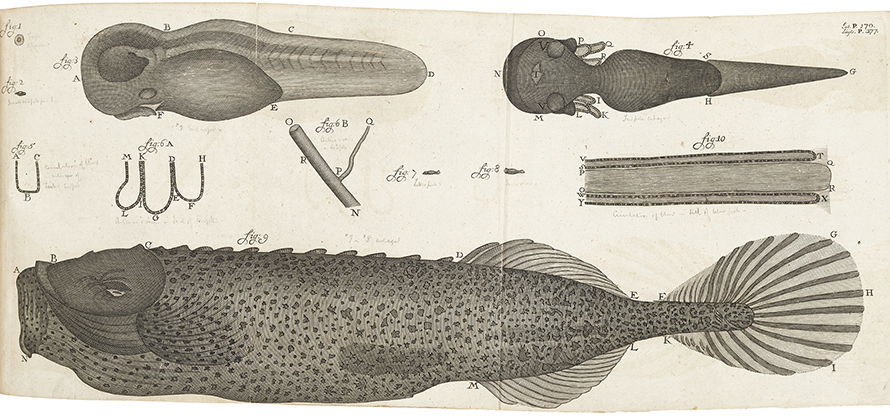
By 1770 the five-step process to prepare tissue for microscopy — fixation (or preservation), processing, embedding, sectioning and staining — was taking shape. English botanist John Hill made the first attempt to fix and harden plant matter for study. Hill softened sticks in a stream, then macerated them in a solution of alum, dried them and submerged them in alcohol.
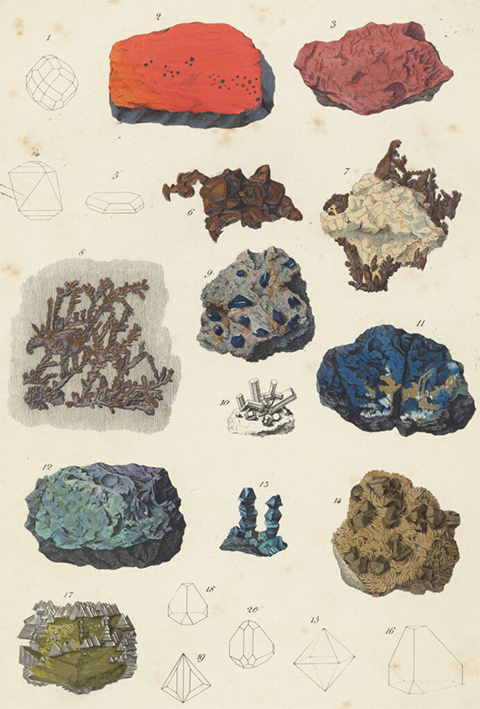
Early histologists often adopted materials long used by artisans and artists to highlight elements of tissue and enhance the contrast among cell parts in their specimens. The commercial availability of carmine made it the preferred dye for the process. Derived from cactus-dwelling scale insects called cochineal, the cultivation of its powerful crimson hue dated back millennia. Nineteenth-century German botanist Theodor Hartig described carmine’s propensity to attach itself to chlorophyll granules, a feature that made it a boon for plant microscopy. Hartig identified other colored substances that could serve a similar purpose, including the yellow gum resin gamboge, the scarlet mercury compound cinnabar and cerulean copper sulfate.
In search of a stain that was both practical and precise, early histologists turned to indigo, gold chloride and the aniline dyes that were just hitting the market in the 1860s. The rich blue-to-purple hue of hematoxylin, an extract from the Central American logwood tree, was particularly good at exposing cell nuclei and gave researchers one of their most potent tools. (It’s the "H" in H&E staining, which remains one of histology’s most ubiquitous tools.)
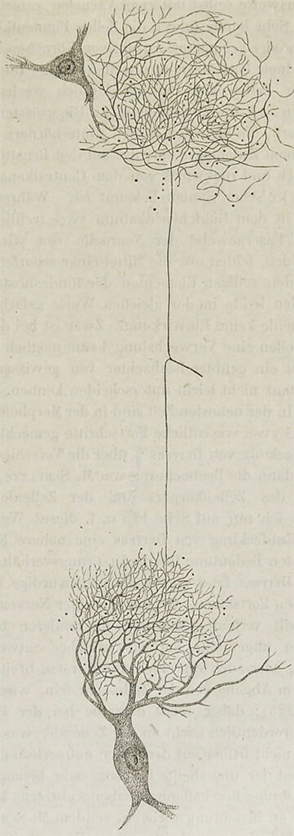
Staining the tissue of the central nervous system is significantly more complex than that of plant matter or even other human tissue. Nerve tissue deteriorates quickly and is densely packed with cells, complicating the task of bringing into adequate contrast each cell’s distinct elements, including the dendrites and axons that extend from the cell body in opposite directions. By leaving a piece of tissue overnight in diluted carmine, the German anatomist Joseph von Gerlach found a way through this impenetrable wall. The extended time in a less concentrated solution finally revealed the differentiation that he and others had been seeking. His 1858 paper on the results demonstrated a level of control over staining technique that encouraged others to try their hand and ignited histological exploration across Europe.
As von Gerlach continued his research, he theorized that nerve cells were connected to one another by a network in which their dendrites and axons branched minutely and endlessly into one another to form a densely interconnected forest. In this continuum, von Gerlach theorized, nerve cells were placed in constant anatomical and functional connection. The reticular theory, as it came to be known — a reticulum being a network or netlike structure — usurped the cell theory promoted by Virchow and others to become the standard explanation for the operation of the brain. In Abbiategrasso, Golgi quickly fell under its spell.
An instrument of revelation
As histology was maturing, a seemingly disparate craft was developing simultaneously that would offer Golgi a tool to gaze into the forest of the central nervous system and see individual trees and their branches for the first time.
Early photographic experimenters in the 18th and 19th centuries noticed the propensity of silver salts to darken when exposed to the sun. By the 1830s, researchers observed that paper bathed first in sodium chloride and then in silver nitrate darkened into shades of varying intensity to reveal the contours of images projected onto it. A rush of technological improvements followed, and crowds and critics were awed by the “divine perfection” of mysterious photographic methods. Artists and scientists, professionals and amateurs of all stripes quickly adopted the tools. Years later, in Spain, a young Cajal found himself “stupefied” by photography and the chemical processes that could conjure a latent image, bringing the seemingly invisible into view.
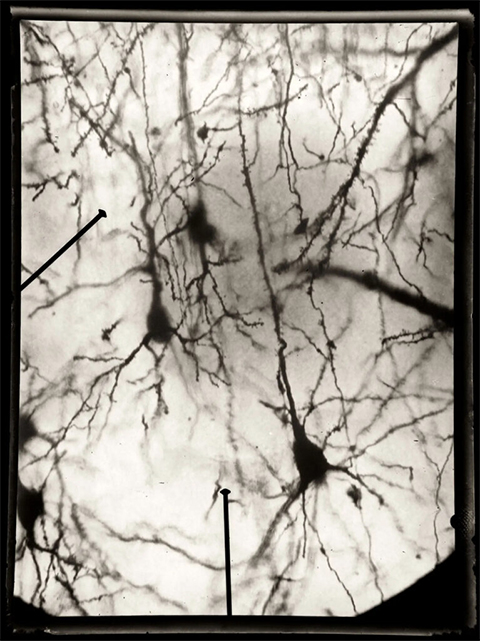
Soon, silver nitrate would be the key ingredient in another scientific breakthrough.
As he poured himself into histological research and experimentation, Golgi focused his attention on the nervous system’s connective substance, what Virchow had called neuroglia, or nerve-cement. Golgi was able to describe their varied shapes — rounded, lenticular, stellate — and attracted international attention for his writings on their relationship to nerve cells. But he recognized the need to move beyond the usual histological techniques in search of those that could “match the special and complex structure” of the nervous system.
Although carmine predominated in the early days of histology, silver nitrate had occasionally been used to stain intercellular substances black. In early 1873, Golgi wrote to a friend to say that he had expanded on this approach by letting silver nitrate react on pieces of brain hardened in potassium dichromate for as long as 45 days. He had “obtained magnificent results and hope(d) to do even better” — magnificent because his concoction mysteriously impregnated only a few cells in a sample with the darkened silver. For reasons that still remain unclear, the black reaction, as Golgi dubbed it, generally stained less than 5% of the microscopic field.
Golgi’s method produced an image with a level of contrast and clarity previously unseen. Where von Gerlach saw trees branching toward one another in a dusky forest, Golgi could now distinguish not just limbs but the finest twigs and shoots of each individual, starkly shadowed against the pale yellow of a morning sky. One of his students later described its “marvelous beauty . . . which allows even the layman to appreciate the images in which the cell silhouette stands out as if it had been drawn by Leonardo da Vinci” and nerve fibers intertwine “with the most sophisticated elegance.”
Armed with this transformative new tool, Golgi began to develop his own version of the reticular theory, which he called the “diffuse neural net.” He insisted that the central nervous system worked as one holistic unit in which electrical impulses are carried throughout by physical connections that bond one nerve cell directly to another. He differed from other reticular theorists by claiming axons alone — and not dendrites, as had previously been suggested — formed the reticulum. Against all evidence that emerged in the years to come, he would remain a prisoner to this idea.
Though Golgi’s black reaction would eventually earn him an invitation to Stockholm, it took more than a decade for the international scientific community to take notice. He published his findings in a journal with limited circulation, and they lacked the illustrations that would have brought the great leap to life. Among the few foreign scientists who immediately recognized the technique’s potential, however, was Cajal, who saw in the “happy peculiarity” of the black reaction’s inexplicable selectivity “the instrument of revelation.”
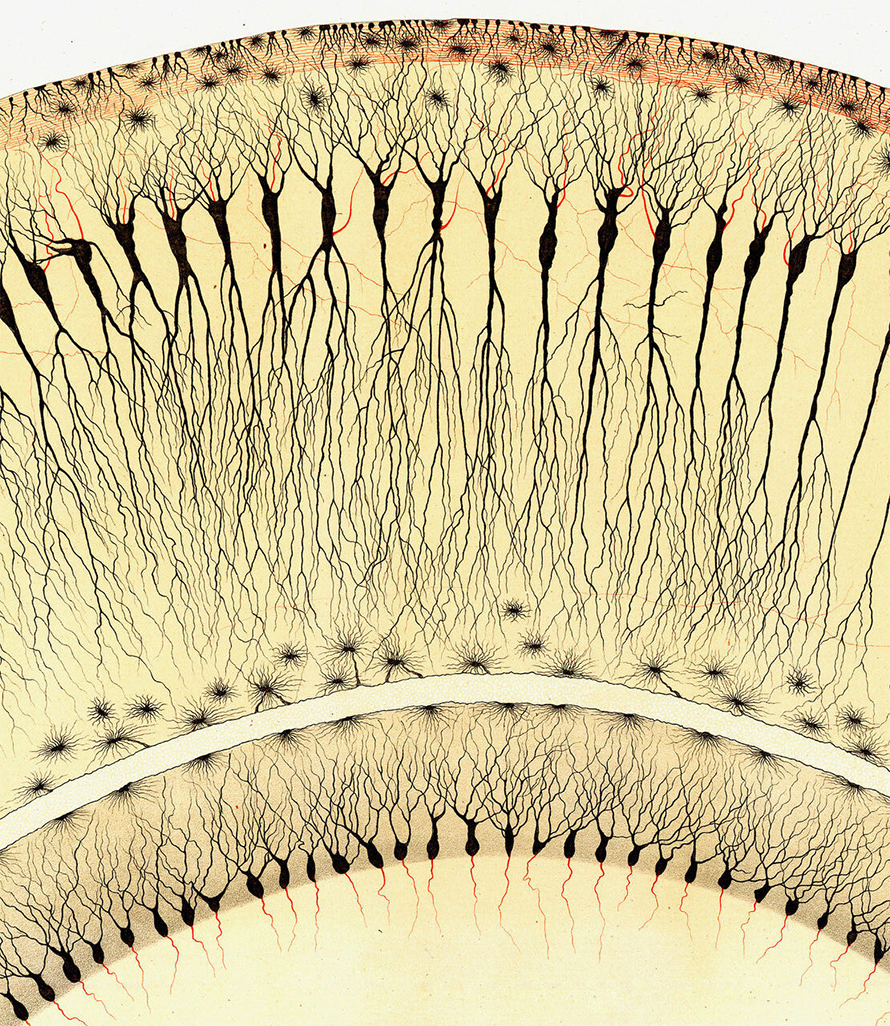
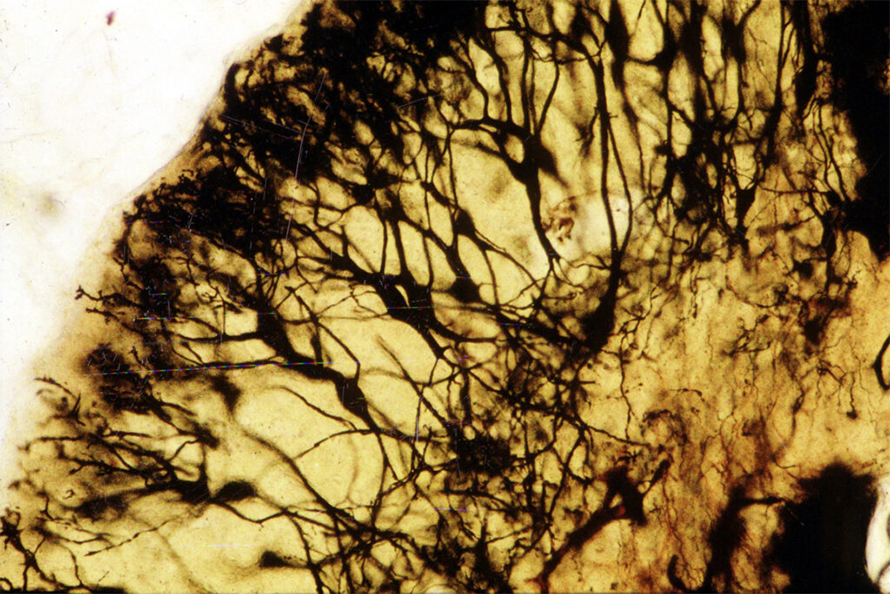
The dream technique
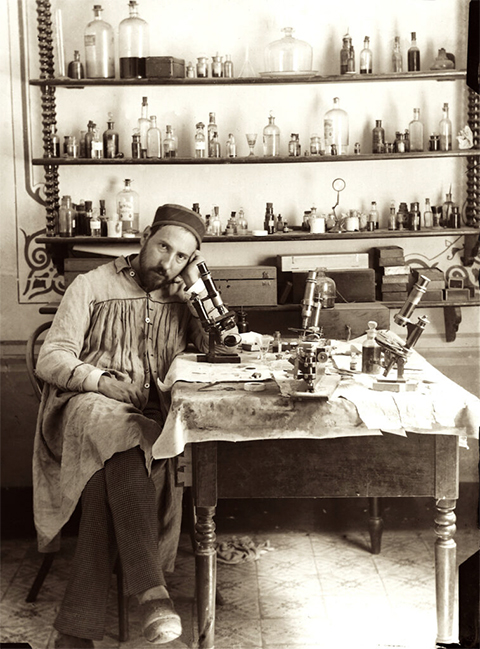
If Golgi’s path to Stockholm was unlikely, his counterpart’s journey was even less predictable. Santiago Ramón y Cajal was born in 1852 in the highlands of the Aragon region of Spain, where the Pyrenees mountains loomed to the north. As with Golgi, the nation of Cajal’s youth was defined by political turmoil — incessant uprisings, government overhauls and rewritten constitutions. Napoleon’s invasion of Spain more than 40 years earlier had driven its economic and political systems to the point of collapse, taking its scientific institutions along with them. Spain had remained a scientific backwater ever since, but Cajal would help resurrect the country’s standing.
As a boy, Cajal was seized by an “irresistible mania” to draw. Every surface he encountered was a canvas. His brother, Pedro, said he “entered the castle of science through the door of art.” For Cajal, art was a way of understanding the world around him. Even from his first microscopic explorations, he drew what he saw in detail, with all the shading and color of the real thing.
Cajal’s father was a barber–surgeon, a centuries-old profession. Since medieval times, the barber’s facility with a blade made him, rather than a physician, the man to see for minor surgeries and bloodletting. At his father’s hand, Cajal learned anatomy through the dissection of cadavers, drawing hundreds of sketches of the structures he and his father uncovered with their scalpels.
The Glorious Revolution of 1868, which ended the tumultuous and controversy-scarred reign of Queen Isabel II, sparked a revival of Spanish science. Translated foreign texts, including Virchow’s book popularizing cell theory, ignited new ways of thinking about the body and medicine, replacing old ideas that posited the soul as the source of healing. For Cajal, the book was a revolution all its own. Like Golgi, he enrolled in his father’s medical alma mater in Zaragoza, Aragon’s capital. There, he saw his first preparations under a microscope. A frog lay paralyzed on its back, its intestines removed, and its lymph sac injected with carmine, staining its cells bright red. As he observed red and white cells rushing through its bloodstream, Cajal found himself deeply moved. “It was as though a veil were suddenly lifting from my soul,” he wrote.
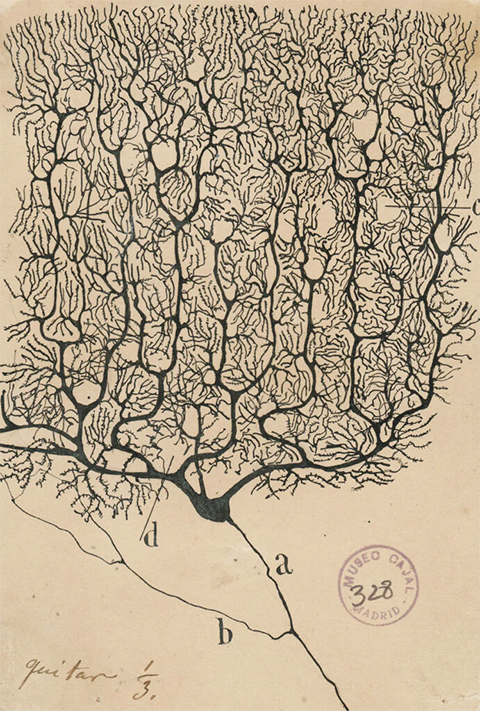
From that point forward, Cajal was inseparable from his microscope. At one point in middle age, during a two-week stay in the London home of neurophysiologist Charles Sherrington, Cajal turned his guest room into a laboratory, refusing to pause his investigations. He and Golgi shared a devotion to their work above all else. “Are there Sundays in nature?” he once wrote, questioning the merits of rest. He and his wife, Silveria, had seven children, but the warmth of family life could not draw him away from research. “Children of the flesh should not drown out the children of the mind,” he wrote.
On his 32nd birthday, in 1884, Cajal published his first textbook, 192 pages detailing for other Spaniards the best contemporary methods for microscopy and staining. It wasn’t until a few years later, though, that he encountered the black reaction. In the lab of a colleague fresh off five years studying with the elite in Paris, Cajal saw preparations of neurons he described as looking like “Chinese ink on transparent Japanese paper.” What had been an “inextricable network” when stained with carmine and hematoxylin was now “simple, clear, and unconfused.” “The dream technique,” he declared, “is a reality!” Within a year of witnessing the black reaction, Cajal had abandoned all other work to study nervous tissue.
Protoplasmic kisses
The question driving Golgi and Cajal’s work was the same: How does the nervous system function? To answer it, they needed to understand the course of an electrical impulse — the path from stimulus to response. A century earlier Italian physician Luigi Galvani had experimented on frogs to discover what he called “animal electricity,” an innate force that activated the body’s nerves and muscles. But how those impulses traveled throughout the nervous system was yet unknown.
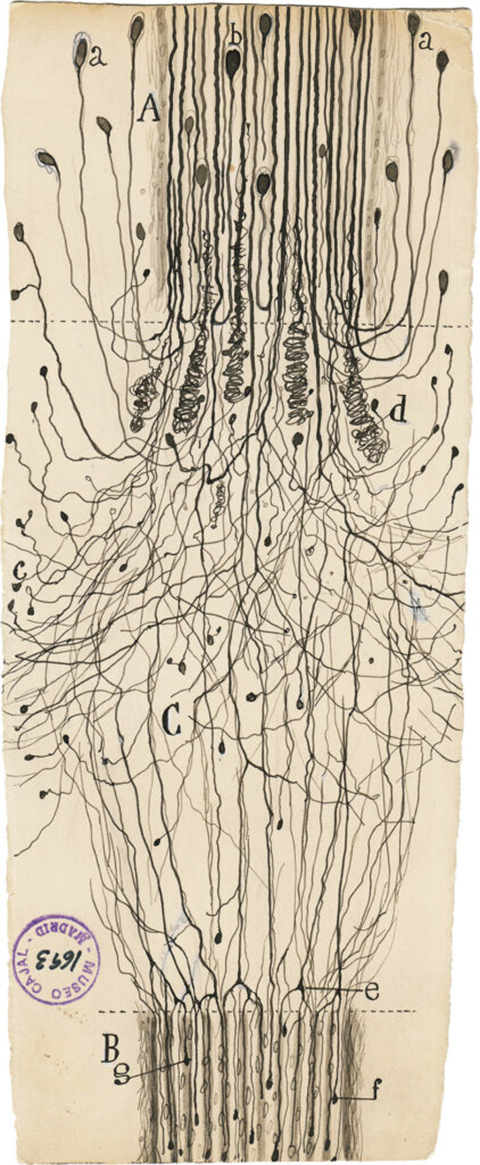
For Golgi, the interconnectedness of the system was its own answer. The reticulum offered “the greatest variety and the greatest complexity” of relationships between nerve fibers, he argued. Cajal, though, wanted to know how the nervous impulse within a nerve cell actually moves: “in all directions, like sound or light or . . . constantly in one direction, like water in a watermill.” Cajal needed incontrovertible proof to believe that nerve fibers maintained a physical connection, and Golgi hadn’t yet offered it.
Cajal’s was a single-minded pursuit: Of the 45 papers he published from 1888 to 1891, every one included the word connections in the title or subtitle. In search of the connections Golgi insisted upon — or the evidence that they didn’t exist — Cajal sought to improve the black reaction’s clarity, staining tissue multiple times with silver nitrate, submerging samples for longer periods of time, or employing more concentrated solutions. Over months of practice and repetition, he reached the level of detail he needed, using a “double impregnation” technique that allowed the stain to penetrate thicker sections of tissue, revealing more about the nerves he was studying. Although he was never able to directly observe the space between neurons, he believed that the absence of color in his preparations represented those gaps. Always, he drew, making nearly 3,000 elegantly detailed sketches over his lifetime of the intricacies of nerve cells and the connections he did and didn’t see.
Under the microscope, Cajal, like Golgi, saw the dense tapestry of the nervous system, a web of ordered chaos, so many spindly roots thinning as they stretched out from a cell in search of connection. But unlike Golgi, he did not deceive himself into believing he saw those connections made real.
In studying the retina and olfactory bulb, a segment of the forebrain that processes odors, Cajal realized that dendrites were oriented toward the external world and axons faced inward toward the body’s nervous organs. He determined that this orientation defined the path of nervous impulses (from dendrites, to the cell body, to axons) and called this the law of dynamic polarization. With his refined staining technique, he was now confident that dendrites and axons both “terminate freely,” refuting Golgi’s argument for physical connection. The impulse is carried across the gap — later described by Sherrington as a synapse — “in much the same way that electric current crosses a splice between two wires,” Cajal wrote. For the Spaniard, who had grown up reading "Don Quixote" and other great literature and wrote fantasy of his own, the inner workings of the nervous system evoked literary romance. Communications between nerve cells were “protoplasmic kisses,” he wrote, “the final ecstasy of an epic love story.”
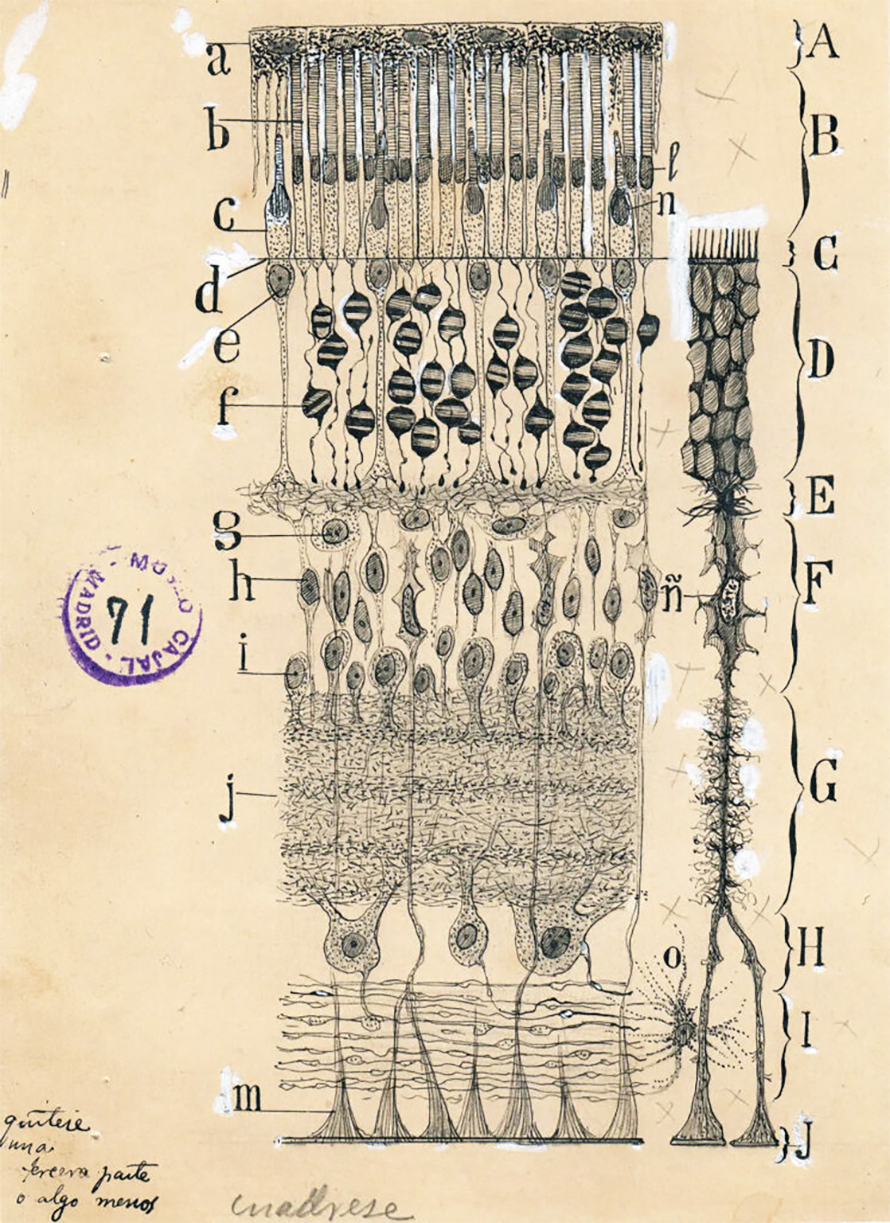
In 1889, he wrote to Golgi to say that he was prepared to present his findings and declare the independence of the nerve cell at an international conference in Berlin. He hoped to visit Golgi in Pavia on his trip home. “My preparations are so clear, so analytical, that all doubts concerning certain facts are absurd,” he told the Italian, who by then had put aside research into the nervous system to focus on infectious disease and was in the process of identifying the parasites responsible for malaria and their relationship to fever. Golgi dismissed Cajal, sending back a paper on his malarial research.
At the conference, Cajal’s colleagues were “enchanted” by what his slides showed, Retzius said, and surprised that such significant research could have emerged from Spain. Many committed to returning home and applying Golgi’s method — perfected, as it was, by Cajal. Golgi had finally been recognized for his staining technique, but it was Cajal’s description of the nervous system’s function that now dominated scientific thinking.
Formidable enemies
Cajal may have professed his admiration for Golgi’s “seminal and path-breaking experiments” and the “precious method” he shared with the world, but he viewed the reticular theory as a “formidable enemy.” Golgi, a man with an “iron will,” a “singular voice” and a “vast and strong forehead,” as one scientist wrote after meeting him, was the theory’s most vocal advocate. To Cajal, whose investigation of nerve cells sometimes veered into psychology, the black reaction had offered a light by which to explore “the utter darkness” of the mechanisms of human behavior. To assert that the entire central nervous system exists in a constant state of connection was to declare “the absolute unsearchability of the soul.”
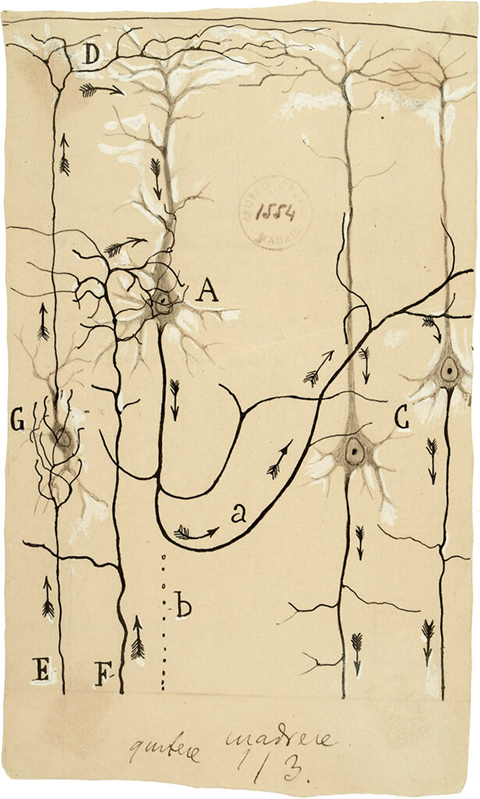
Following the conference in Berlin, the scientific community coalesced around Cajal’s theory of the individuality of nerve cells, which in 1891 was described by German anatomist Wilhelm von Waldeyer as the neuron doctrine — neuron referring, in the original Greek, to sinew and nerve, among other objects. (Waldeyer also coined the term "chromosome.")
Despite the shifting tide, Golgi stubbornly continued to fight for his theoretical interpretation. As he approached his 50s, his chestnut hair beginning to gray and his prodigious forehead expanding, his interest in nerves was reignited by the “strenuous opposition” his hypothesis had attracted, as he detailed in a paper attacking the neuron theory and the law of dynamic polarization. Those who disagreed with him — Cajal, most notably — were concerned more with “doctrinal conceptions than on new proven facts,” he wrote. “I must ask if this is really doing anatomy or rather exercising imagination.” Even the word "neuron" itself, he said, “cannot claim any well-grounded right to citizenship in science.” Cajal, meanwhile, lamented reticular theory as a “contagion” and its adherents as “fanatics” driven by “an anarchical and calamitous passion.”
At conferences, in journals, in lectures and in correspondence with other scientists, the rivals waged ideological war. Still, as they pushed forward in their research, they seemed to orbit one another. Cajal did visit Pavia on his return from Berlin in 1889, but Golgi wasn’t there, and the chance to bridge the gap between the histologists was lost. Five years later, both men spoke at an international conference in Rome, along with Virchow, a shared inspiration. Cajal discussed the morphology of nerve cells, while Golgi served on the conference’s executive committee. But Cajal’s meticulous memoir makes no mention of them meeting.
Still, they remained entangled. When Cajal published his discovery of delicate fibers, which he called “collaterals,” branching from axons in the spinal cord of chicken embryos, Golgi was incensed. A decade earlier he had made the same finding, but it had gone unnoticed in the provincial Italian journal that published it. Golgi’s subsequent discovery of the organelle that now bears his name provided a bit of symmetrical irony. He found a “fine and elegant reticulum hidden in the cell body” in 1897 — several years after Cajal had obtained images of a similar structure in pieces of a young rabbit brain but opted against publishing the observation because he was unable to reproduce his finding.
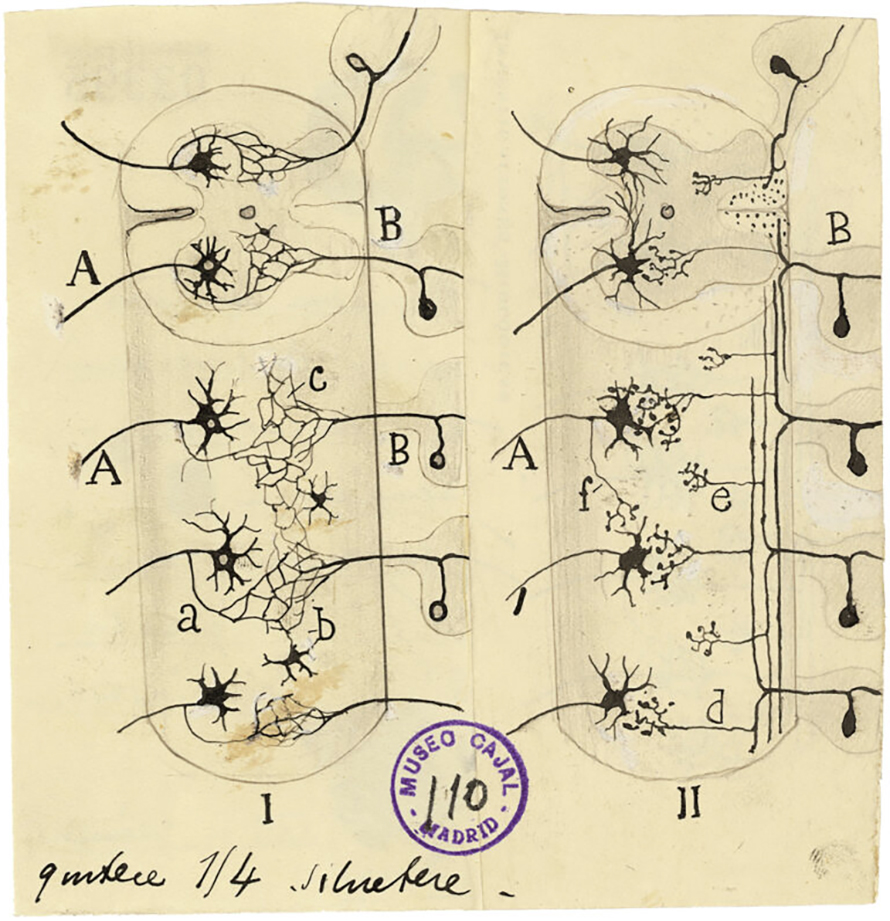
In 1903, Cajal vacationed in Italy and once again made a stop in Pavia; Golgi, however, was on his own vacation elsewhere. While taking photographs in Rome, Cajal conceived of yet another new staining technique, reducing silver nitrate with the developing agent pyrogallic acid to create photosensitive compounds that would blacken upon exposure to light, revealing even more about the inner structure of nerve cells. It allowed him to prove that nerve fibers form only within cells and not between them — a final nail in the coffin of reticular theory.
Golgi, however, would not relent. Three years later, the Nobel committee made the controversial decision to split the prize between him and Cajal, despite Golgi’s primary discovery being now long in the past and his conceptual framework giving way to Cajal’s. Golgi, who believed the Spaniard was ill and unable to travel to Stockholm, was stunned to emerge from his train and see Cajal among those waiting to welcome him, insistent upon finally meeting his rival. In the biting Scandinavian air lay one last chance to break the ice between them. Instead, Golgi stormed to his hotel to revise and rehearse the Nobel lecture that might wrest back his scientific authority.
A relic of the past
At the Nobel ceremony on Dec. 10, the president of the institute administering the award praised Golgi as the “pioneer of modern research in the nervous system” and Cajal as the man who had “given the study of the nervous system the form that it has taken in the present day.” But when Golgi stepped up to deliver the prizewinner’s customary address at noon on the 11th, he dispensed with any such harmony. The audience was stunned as he launched into an attack on the neuron doctrine that betrayed his bitterness at seeing his own work turned against him.
Rather than expounding his own findings, as was typical in such a lecture, Golgi claimed that the neuron doctrine was “generally recognized to be going out of favor.” He lamented that he was “unable to follow the current of opinion” that declared the “so-called physiological independence of the neuron,” and he scoffed at Cajal’s law of dynamic polarization. He saw no reason to give up his concept that nerve cells acted together, rather than individually. “I cannot abandon the idea of a unitary action of the nervous system,” Golgi said. He didn’t care if his stubborn belief tied him to a theory others had cast aside.
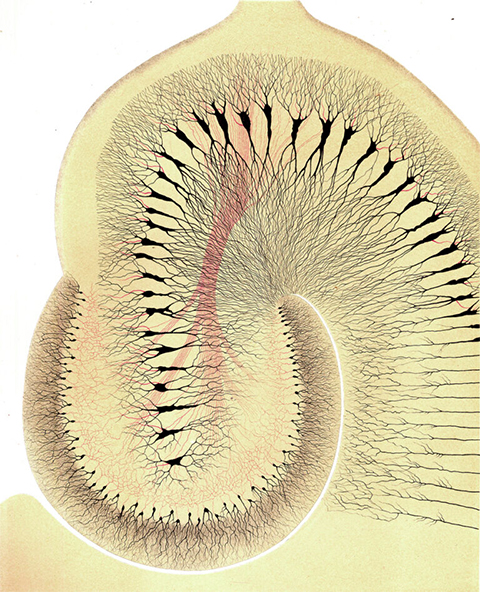
After so many years spent lobbing scientific salvos at one another, the mild-mannered Golgi had taken aim directly at Cajal — and, in the process, ignored or diminished the contributions of many of the scientists, including Retzius, who had shared in his explorations and achievements. Cajal, whose work Golgi subtly critiqued as “frequently plausible,” joined members of the audience who watched in “stupefaction,” he later wrote. Cajal recalled “trembling with impatience” and being desperate to intervene, wishing to correct his rival’s “odious errors” and “deliberate omissions.” He wanted to put the past in its place and turn the page toward progress.
At his own lecture the following day, Cajal gave a more traditional address with that aim in mind. But he allowed himself one parting shot. “It would be very convenient and very economical” if nerve cells truly did form a continuous network, as Golgi argued. “Unfortunately,” Cajal said, “nature seems unaware of our intellectual need for convenience and unity, and very often takes delight in complication and diversity.”
The cruel irony of fate
Golgi and Cajal continued a distant scientific exchange of sorts after Stockholm. Golgi returned to studying the internal reticular apparatus and found an improved method for staining it, using a modification of Cajal’s reduced silver nitrate technique. Despite his varied contributions, his scientific standing never recovered. The second volume of Cajal’s memoir, "Recollections of My Life," published in 1917, offered harsh attacks on Golgi that became the basis for his international reputation in the years to come. Even in Pavia, Golgi became known not for his discoveries but for his errors. Rather than the forefather of neuroscience, he was the man who let pride stand in the way of progress.
Cajal, meanwhile, developed two more histological techniques to better reveal neuroglia, contributing to researchers’ realization that beyond neurons lay an assortment of cells in the nervous system more diverse than they had previously grasped. As he grew older, he was preceded everywhere he went by the fame he had achieved for his discoveries and that had been cemented with the Nobel. Bands played for him in public, students applauded at the sight of him, and statues went up while he still lived. He became a monument to Spain’s scientific might. In 1956, Sanford Palay, an American neuroscientist, used the electron microscope to produce the first images of a synapse. He wrote that it confirmed Cajal’s theory, referencing him by name.
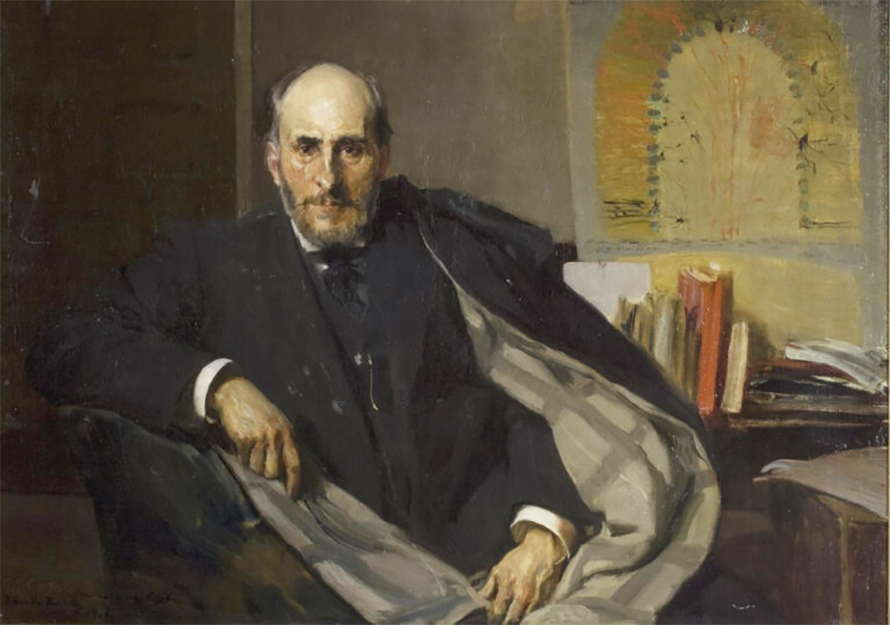
Like two neurons, Golgi and Cajal stood on opposite sides of a chasm, a synapse that separated them as they sought to perceive what had forever been invisible. Despite this gulf, a kind of current passed between the two rivals, a stimulus and response that carried neuroscience into a new era. From their feud emerged the technical tools to explore the brain’s building blocks and the theoretical framework by which to understand its function.
“What a cruel irony of fate,” Cajal wrote after the Nobel lectures, “to pair, like Siamese twins united by the shoulders, scientific adversaries of such contrasting character!”
Originally published as “A Cold Day in Stockholm” in Distillations Magazine, courtesy Science History Institute.

Enjoy reading ASBMB Today?
Become a member to receive the print edition four times a year and the digital edition monthly.
Learn moreGet the latest from ASBMB Today
Enter your email address, and we’ll send you a weekly email with recent articles, interviews and more.
Latest in Science
Science highlights or most popular articles
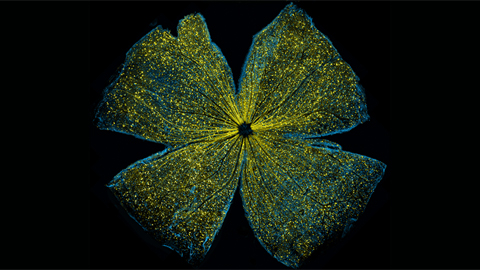
Calcium channel linked to cancer drug resistance
Researchers discover a protein associated with carboplatin-resistant retinoblastoma, suggesting this protein could be a promising therapeutic target. Read more about this recent Journal of Biological Chemistry paper.
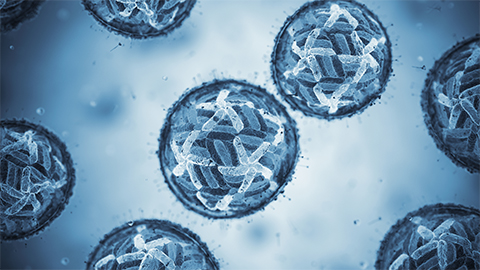
Host fatty acids enhance dengue virus infectivity
Researchers in Germany find that viral replication depends on host enzymes that synthesize lipids, revealing potential metabolic targets for antiviral intervention. Read more about this recent Journal of Biological Chemistry paper.
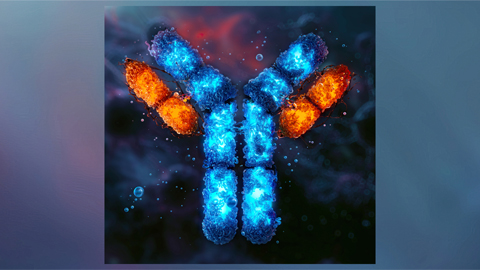
Antibodies inhibit hyperactive protein disposal
Researchers at the University of California, San Francisco, identify an enzyme inhibitor, offering new tools to study diseases like cystic fibrosis, neurodegeneration and cancer. Read more about this recent Journal of Biological Chemistry paper.
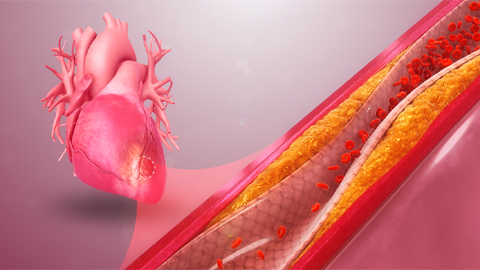
Scientists find unexpected correlation between age and HDL-C levels
In a 30-year multicenter study, researchers determined what factors predict HDL-C concentration. In their analysis, they found that HDL-C levels grew with increasing age and physical activity.
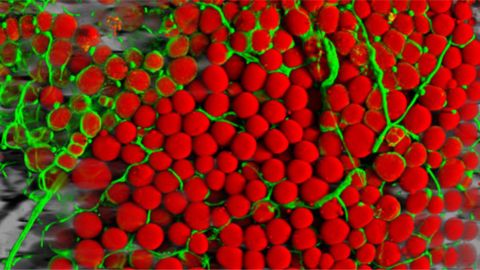
Butter, olive oil, coconut oil — what to choose?
Depending on the chain length and origin of the fat, regular fat consumption changes the specific makeup of fats in bloodstream and affect mild to severe cholesterol patterns. Read about this recent Journal of Lipid Research study.
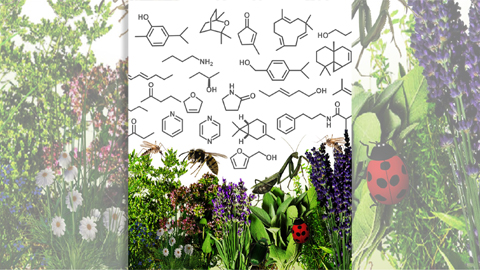
Computational tool helps scientists create novel bug sprays
Rapid discovery of mosquito repellent compounds is enabled through a novel screening platform that combines both computational modeling and functional screening.