The slime and grime that stick to ships
When a barnacle wants to stick to something, it opens up a capillary and bleeds. The sticky torrent of enzymes and fibrous tissues that follows gloms onto a ship hull, dock or any other submerged surface and calcifies into a permanent scab. While this is great for the barnacle, who now has a home and can begin seriously to consider starting a family, it’s bothersome for a ship’s operators, as the growing masses of sea creatures stuck to the vessel add drag, increase wear and tear on the ships and make trips more expensive.
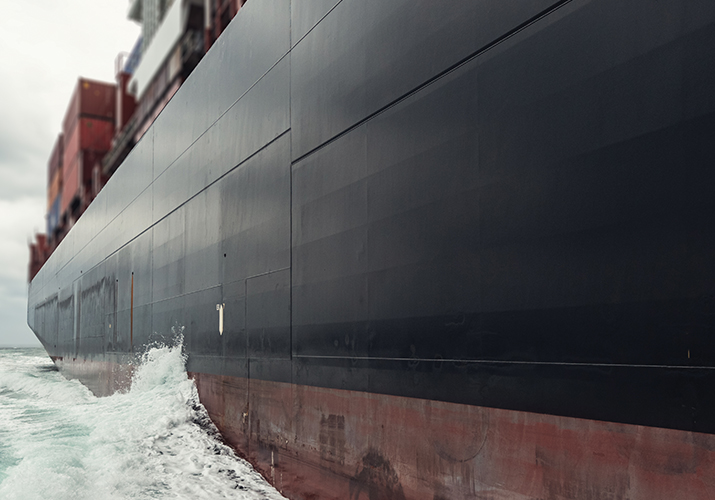
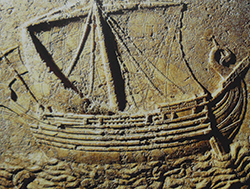
This phenomenon, known as biofouling, has addled ships for as long as humans have roamed the seas. The ancient Phoenicians, one of the earliest seafaring societies, reportedly coated the bottoms of their tublike ships with pitch or copper to repel the organisms that liked to colonize them. Ship design has evolved greatly over the millennia, but biofouling organisms remain a significant problem even in the age of supercarriers and stealth destroyers. A study published in 2010 estimated the annual cost of biofouling to the entire U.S. Navy to range from $180 million to $260 million.
There are two dominant methods for handling biofouling organisms: kill the adhering microorganisms with antifouling coatings before they can settle or provide a foul-release surface that they’ll slide off once the vessel is operating at high speed. However, each comes with its own drawbacks. To overcome the shortcomings of foul-release and antifouling coatings, a third variety of coatings, which exploit the biochemistry that fouling organisms use to stick to hulls, are being developed. But experimental coatings can be difficult to assess in the dynamic environment of the ocean; even the most promising coatings being tested in laboratories are leagues away from making their way to the ship hulls being colonized in thousands of ports worldwide.
So, in the meantime, fouling is an inevitability.
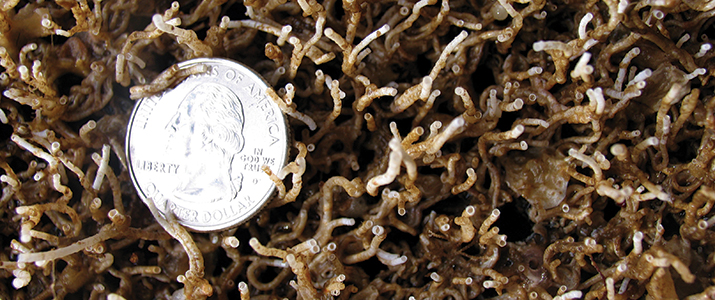
First contact
Barnacles and tubeworms are some of the most prominent and visible biofouling organisms that gather on marine surfaces, but the sticky microbial films that they share space with almost always beat them to the hulls.
“When a new surface is put in the water — for example, a brand-new, newly painted ship — it’s colonized by microbes very rapidly,” says Benjamin Van Mooy, a chemical oceanographer at Woods Hole Oceanographic Institution. Once attached, the microbes quickly establish a slimelike coating. “That’s like a green, slippery film, and it’s all made of microbes,” he says.
Like many biofilms, the slime provides a matrix for signaling and other interactions among bacteria, and it is held in place by a sticky, transparent polysaccharide glue. But because of the diversity of bacterial species in the film, not all of those interactions are in the best interest of a bacterium’s neighbors.
“For every group of organisms that’s trying to get a competitive advantage by signaling and coordinating by signaling, there’s also another group of organisms that’s trying to get a competitive advantage by disrupting the signaling of their competitors,” explains Van Mooy.
The members of that bacterial community, which can include photosynthetic cyanobacteria, diatoms and other heterotrophic bacteria, all have evolved adaptations for a relatively stationary life on a surface. “They’re not the same microbes that are floating around in the water, so it’s not like the ship hull is flypaper,” says Van Mooy.
The slime coatings are “the Achilles heel of antifouling and fouling-release coatings,” says Mike Schultz, professor and director of the hydrodynamic laboratory at the U.S. Naval Academy. Even if a coating can shear organisms off at 10 knots, it doesn’t do much good when a ship is in port for months at a time, which is standard operating procedure in ports from Norfolk, Virginia, to Oahu, Hawaii.
Teeming with tubules
Hyroides elegans is a tubeworm that would love to settle down on the underside of a ship’s hull. In their mature form, the tubeworms, also known as hydroides, form tubes made of calcium carbonate that are a major contributor to biofouling in temperate waters around the world.
According to Michael Hadfield, a professor at the University of Hawaii at Mānoa, these calcareous “squiggly tubes” beleaguer dock workers tasked with maintaining the ships at Pearl Harbor. He says that port workers report the tubes “build up on the big propellers of an aircraft carrier a layer a couple of centimeters deep in a matter of one to two months.”
The tubeworms have a quick lifecycle and are capable of settling down to begin developing their crusty tubes within five days of hatching from an egg. The metamorphosis from a free-swimming filamentous larvae to a stationary, plume-crested adult begins when a tubeworm is jabbed by a nanometer-scale harpoon fired by Pseudoalteromonas luteoviolacea, a bacterium sometimes found in marine biofilms.
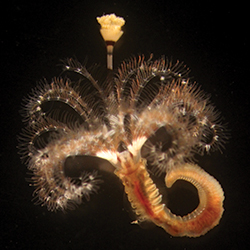
Once hit by a bacterial harpoon, a tubeworm quickly secretes a mucus strand to tether itself to the surface, followed by a primary tube made up of proteins and fibers. Within this tube, the tubeworms undergo metamorphosis and swap out the bands of cilia that had allowed them to swim and feed for a large plume of tentacles. After completing metamorphosis, the worms build a calcified tube that sticks perpendicularly to the surface of the ship or dock.
If the juvenile tubeworms are unable to find a surface, they can remain as larvae for seven weeks or longer. This relative longevity is essential, as the interactions between hydroides and the harpooning bacteria occur by chance, in the complete absence of signaling molecules from the bacteria.
The lack of signaling molecules wasn’t confirmed until Hadfield and his colleagues published a paper in Science in 2014. Up to that point, “people were under the impression that bacteria were just producing passive molecules,” says Nicholas Shikuma, an assistant professor at San Diego State University who was a co-author on that paper. “We figured out that the bacteria were producing these very complex structures that are syringelike,” says Shikuma. He and colleagues hypothesize that these nanometer-scale syringes poke the tubeworm larvae and act as “the stimulant for settlement and metamorphosis.”
While P. luteoviolacea isn’t the only bacteria that can induce hydroides to undergo metamorphosis, it is the only bacteria known to use the syringe mechanism. In the journal Scientific Reports, Hadfield and his colleagues recently described the mechanisms that other common members of marine biofilms use to spur hydroides metamorphosis.
Regardless of which bacteria help to initiate it, the entire metamorphosis process for the tubeworms takes less than 12 hours, and before long the mature tubeworms are ready to start reproducing again. Under ideal circumstances, the turnaround time for a generation is about three weeks. The worms are prolific breeders too. “The females will spawn over and over, and they spawn about 2,000 eggs every time they do,” says Hadfield. “They’re little reproductive machines.”
Combined with the surge in global shipping routes, this has made the tubeworms’ presence both ubiquitous and genetically indistinct in major ports around the world.
“This sort of warm-water biofouling community is just about uniform all the way around the world,” says Hadfield. “These organisms have been spread by ship traffic around the world since the late 1400s.”
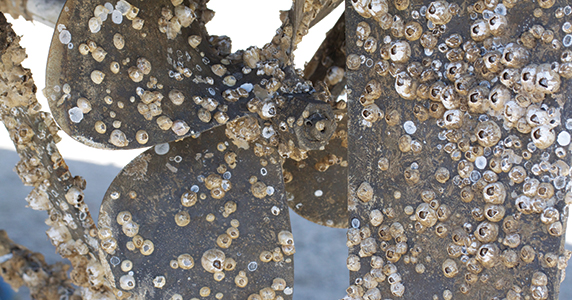
Blistering, bleeding barnacles
Barnacles are arguably the organisms most associated with biofouling, in part because the hundreds of different species colonize a wide variety of marine surfaces, ranging from rocks and docks to whale fins. Their interior base and stalks are hidden by hard, calcified plates and encircled by a cuticle made of chitin.
Barnacles begin their lives as one-eyed larvae that consist of a head and tail. The barnacles swim, eat and pass through a series of five molting phases, winding up as antennae-wielding larvae in search of homes.
During this exploration process, the barnacles produce an adhesion protein that alerts them to a suitable surface. Once a surface has been found, “the larvae will stop exploring, settle and send out signals to attract other larvae,” says Julius Vancso at the University of Twente in The Netherlands. “Then, a colonization of the surface starts.”
After a barnacle has settled, it will eventually grow and molt, producing a new cuticle in a manner similar to an insect producing a new exoskeleton. As this cuticle widens, it cuts into both the underlying surface and the barnacle, causing the crustacean to bleed into the space below. The blood forms a clot, which sticks to the underlying surface. As the molting continues, the barnacle also forms open-ended capillaries whose secretions spot-weld the crustacean in place to the surface beneath.
While the blood is flowing, “all the enzymes you can imagine in your blood clotting system are there,” says Daniel Rittschof at Duke University, who helped pioneer models for studying barnacle growth. This immune response helps to wipe out the bacterial populations near the barnacle. Some species of barnacle also calcify the area beneath them to stick more firmly in place.
“It’s like you’ve cut yourself. You’ve got a scab, and your body reworks underneath that scab,” says Rittschof. “In the barnacles, the scab never falls off.”
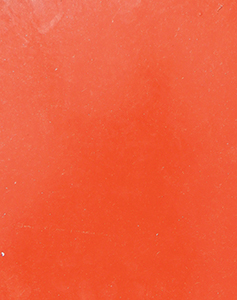
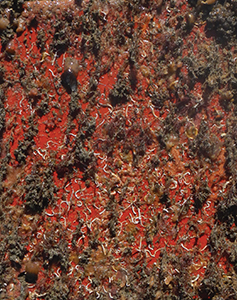
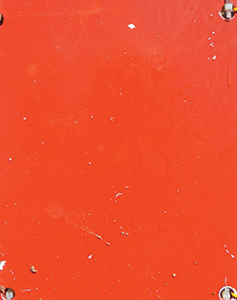
Kill or release
The global uptick in ocean travel following the 15th century spurred innovation for keeping ships free from biofouling organisms. Around the mid-18th century, the pitch-based mixtures that had been the most widely used coatings were supplanted by copper as the official standard for the United Kingdom’s Royal Navy.
According to Stephen McElvany, the program officer overseeing the Office of Naval Research’s antifouling and fouling release coatings program, the U.S. Navy uses two primary types of coatings, which are copper oxide-based ablatives and silicone-based foul-release coatings.
The copper-oxide coating is a remnant of the older way of tackling biofouling that Rittschof calls “mix and kill.” In this method, biocidal compounds that leach into the water surrounding the ship are mixed into the paints applied to the hull.
Copper primarily works by poisoning metabolic pathways, such as photosynthesis, says Van Mooy. The pathway is essential for the cyanobacteria and diatoms that make up a marine biofilm. On commercial vessels, this approach reached an apex of sorts with organotins, which are compounds based on tin linked with hydrocarbons.
At concentrations on the microgram level, organotins, which were highly effective at keeping hulls clean, work by interfering with an organism’s energy production. However, at thousand-fold lower concentrations, the compounds cause female mollusks to transform into males, which had devastating effects on the French oyster fishery in Archaron Bay in the 1970s. The compounds, which weren’t ever implemented in U.S. Navy ships, were banned in 2001 by the International Maritime Organization’s International Convention on the Control of Harmful Anti-fouling Systems on Ships.
The less harmful silicone coatings, which are used in both military and commercial vessels, are rubbery and similar to caulk coatings in our homes.
“It’s not exactly like your bathtub, but what happens then is the fouling that gets on there is easy to peel off,” says McElvany. “If a barnacle sits on there, you can just peel it off easier than (if it were on) a steel surface or a copper paint surface.” Once a ship leaves port and hits speeds of 10 to 20 knots, he says, the hydrodynamic motion of the water against the ship hull shears off the film and crust.
While they’re more environmentally friendly than the copper ablative coatings, silicone paints are more expensive and less durable. When some species of barnacles move in and the surfaces get scratched, the easy-release properties diminish.
“Barnacles are famous for digging under softer coatings and getting a grip that way,” says Anne Meyer at the University of Buffalo. “They actually cut into the coatings.”
However, if barnacles can be prevented from attaching altogether, the cutting and glomming processes can’t damage coatings. Working with colleagues at the Agency for Science, Technology and Research in Singapore, which is known as A*STAR, Vancso used atomic force microscopy to determine the isoelectric point of the adhesion protein that barnacles use to stick to surfaces.
The researchers can use this information to engineer a surface that exploits electrostatic attraction to interfere with barnacle adhesion. “We’ve reasoned that if we can control the adhesion between this adhesion protein … and eventually prevent that adhesion, then these larvae would explore, see nothing and then die, because they stay alive in the metamorphic cycle for a couple of days,” says Vancso.
This type of surface and the experimental coatings being pursued by the Office of Naval Research both belong to a third category of coatings that seek to make ship surfaces essentially invisible to the organisms by exploiting their biochemistry.
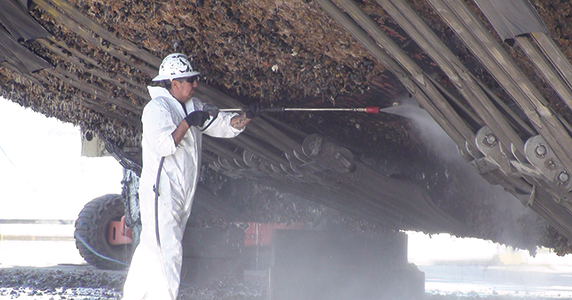
Scenes inside tubes
Biofouling is also a problem in the coolant pipes of a ship’s engine. “If you get too much barnacle growth in your inlet pipes, then you can restrict the flow of coolant, which will limit how fast you can run until you can clean it out,” says Vic Raines, a chief engineer with American President Lines, an international container shipping and ocean freight provider.
The primary method of dealing with clogged engine tubes is overengineering. Raines gives the ship’s cooling system as an example. “Cooling is one of those things that only really matters when you run out of it,” he says. Through overengineering, or building more pipes than the engine requires to be cooled at its highest speeds, the cooling system can afford to lose efficiency due to barnacles choking off pipes.
Other methods of dealing with fouling organisms in the coolant pipes include running electrical currents through copper plates to release ions and using electrical currents to generate sodium hypochlorite, also known as bleach, from the oxygen and sodium chloride in sea water.
According to McElvany, these experimental coatings include polymers that are amphiphilic, containing both hydrophobic and hydrophilic entities on their polymer chains. These each repel certain swaths of fouling organisms. Other coatings involve zwitterions, which cause a positive and negative charge separation at a distance of two or three carbon atoms. This charge separation creates a highly ordered water layer at the paint surface due to hydrogen bonding with the water molecules, which prevents organisms, such as barnacles, from sensing and settling on the hull.
But the experimental coatings are just that, for the time being. The coating that exploits isoelectric points developed by Vancso’s colleagues at A*STAR has been tested only on small substrates under controlled laboratory conditions. “It is not uncommon for the technology to take decades, 10 or 20 years, to develop,” says Anbanandam Parthiban, a colleague of Vancso’s at A*STAR.
For one or all
Biofouling continues to exact a cost on vessels in a number of ways. (See box “Scenes inside tubes.”) “Mariners have been faced with ship-hull fouling since they went to sea, and so it just kind of goes to show what an incredibly enduring problem this is, that it’s persisted for centuries,” says Van Mooy, who is exploring antifouling solutions for deep-sea sensors used in scientific research.
“Various antifouling strategies have been tried, but there’s been no magic bullet,” he says. “I wouldn’t say ‘boutique antifouling’ methods, but I think that certain types of shipping are going to have to have certain types of fouling-release and antifouling formulations that are best suited to what they’re doing.”
Like many of his colleagues, Vansco is concerned about the matter of specificity versus targeting all biofouling organisms with a single coating in the future.
“The next question is, all right, we’ve prevented attachment of barnacles, which is an important fouling species, but what about other fouling species?” says Vancso. “Can we ever have a generic recipe for a universal coating which, if you would apply to the hull of a ship, would prevent attachment of all these fouling species? And that’s a big question that still needs some answering.”
Enjoy reading ASBMB Today?
Become a member to receive the print edition monthly and the digital edition weekly.
Learn moreGet the latest from ASBMB Today
Enter your email address, and we’ll send you a weekly email with recent articles, interviews and more.
Latest in Science
Science highlights or most popular articles
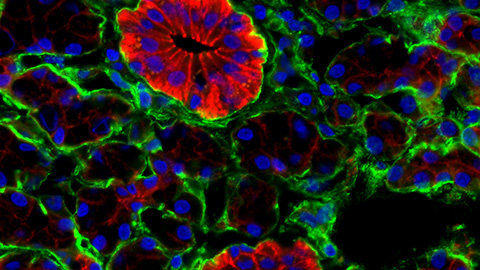
The quest to treat and cure xerostomia
Blake Warner, chief of the Salivary Disorders Unit at the NIH talks about his lab’s efforts to develop treatments for dry mouth.
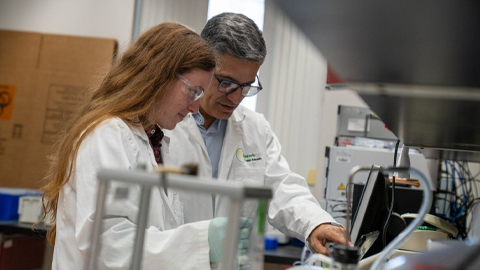
There's more to blue cheese than just the stench
Virginia Tech researchers discovered a way to synthesize a compound in the mold of blue cheese that has antibacterial and anticancer properties.
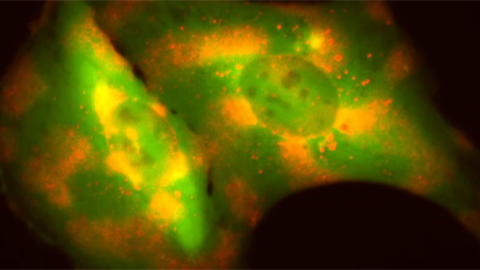
Engineering cells to broadcast their behavior can help scientists study their inner workings
Researchers can use waves to transmit signals from the invisible processes and dynamics underlying how cells make decisions.
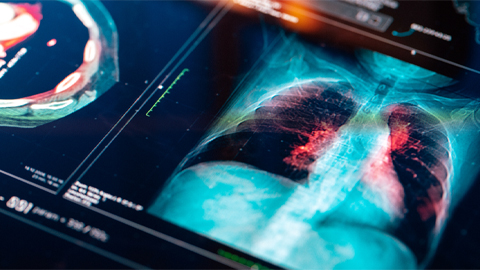
From the journals: JBC
Lung cancer cells resist ferroptosis. ORMDL3 in ulcerative colitis. Novel genetic variants in thyroid cancer. Read about these recent papers.
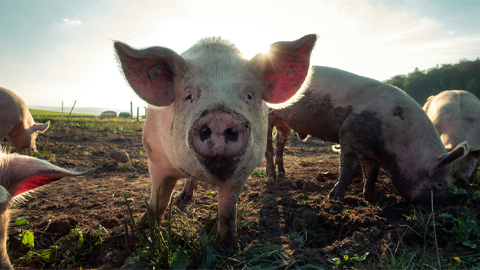
Seeking the sweet spot to beat a pig parasite
Researchers extracted, separated and tested glycans from the porcine whipworm in an effort to determine the best way to develop treatments and vaccines.
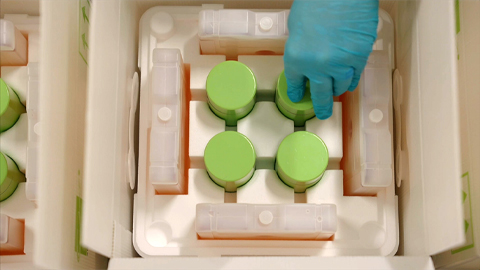
Radioactive drugs strike cancer with precision
The tumor-seeking radiopharmaceuticals are charting a new course in oncology, with promise for targeted treatments with fewer side effects.