The time of trials: Waiting for a coronavirus vaccine
The COVID-19 coronavirus has knocked our world off its axis. We won’t return to anything approaching normal — that is, life without social distancing, quarantines, masks, school closures and other control measures — until most of the world has been vaccinated against the virus. Everyone, therefore, has the same question on their mind: How fast will a vaccine be ready?
The history of vaccine development is not encouraging. “I’ve been working on vaccines for a long time,” says Barney Graham, deputy director of the Vaccine Research Center at the US National Institute of Allergy and Infectious Diseases. “I’ve never seen one take less than about 20 years.” It took 26 years to develop a vaccine for the human papilloma virus, for instance, and 25 years to secure one for rotavirus. And researchers have been trying for more than 50 years to find a vaccine against respiratory syncytial virus, one of the leading causes of infectious disease mortality in infants. Even after Graham’s group figured out a better approach in 2013, the vaccine is still only in the testing phase.
These are not normal times, however, and a vaccine for the COVID-19 virus, formally known as SARS-CoV-2, is the focus of unprecedented research efforts. Already, over 100 research groups have vaccine candidates under development, and a few are already being tested in people. In mid-May, the US government announced “Operation Warp Speed,” an initiative that aims to have a vaccine ready for general use by the end of 2020.
Almost all experts say that target is too optimistic, generally citing the spring of 2021 as a best-case scenario. But to hit even that later target, a lot of things have to break right, and a lot of logistic hurdles have to be cleared away. Here’s a look at some of the key issues in vaccine development.
What kinds of vaccines are there?
All vaccines aim for the same goal: exposing the body’s immune system to protein or carbohydrate fragments, or antigens, displayed by a virus or other pathogen. If all goes as planned, “memory cells” within the immune system remember this introduction. If the vaccinated person is later exposed to the actual virus, these cells enable the immune system to react quickly, suppressing the disease or reducing its severity.
Where vaccines differ is in how they present those antigens. Some vaccines, such as ones against measles and polio, use the entire virus that has been either killed or damaged so that it no longer causes disease. Because these vaccines use the whole virus, researchers don’t need to know as much about the virus and its proteins. But because a whole virus offers many antigens to the immune system — and because of the slight risk that a live virus could become pathogenic again — more can go wrong. So whole-virus vaccines need extensive safety testing, a process that can take years.
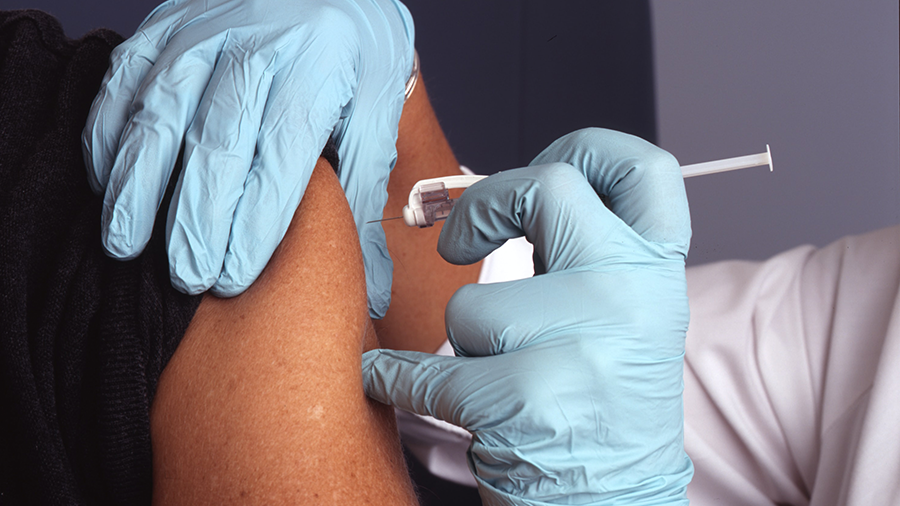
Other vaccines extract the viral gene that codes for the desired antigen and insert it into another, less harmful virus that is then delivered to the patient (the recently approved vaccine for Ebola is a case in point). Still others use bacteria or yeast to manufacture the antigen in fermentation vats. The antigen can then be injected directly, as in the hepatitis B vaccine, or used to build empty shells of viruses that lack genetic material, as in the vaccine against human papilloma virus.
Newer, more experimental vaccines are on the table too. They deliver not the antigen itself but the genetic material that codes for it, either as RNA or DNA, usually encapsulated in a fatty membrane. This enters the body’s cells and directs them to make the relevant protein themselves to trigger the immune response. Such vaccines could be quicker to create because genetic material is easier to mass-produce than proteins are. But RNA and DNA vaccines are so new that none have yet been approved for use by the general public.
Why does it take so long to develop a vaccine?
Before a vaccine is ready for public use, researchers must prove to government regulators that it is both effective and safe to use. That takes time.
Like all medicines, after vaccines are tested in experimental animals, they go through three phases of testing in people. First, a few healthy volunteers receive the vaccine: This Phase 1 trial tests for safety and gives a rough idea of how much vaccine is needed. After that, researchers work out dosing and safety in more detail in a somewhat larger group — the Phase 2 trial.
These preliminaries can be dealt with in a few months, if all goes well. But before a vaccine can be approved for general use, it must be given to a much larger group and compared with an unvaccinated control group, to see whether it really prevents disease. This Phase 3 trial is the most time-consuming step in testing, because researchers have to wait for enough participants to be exposed to a virus naturally. “You cannot compress time when you’re relying on a natural exposure to occur,” says Michael Yeaman, an infectious disease specialist at UCLA and coauthor of a 2017 overview on vaccines in the Annual Review of Pharmacology and Toxicology.
Building manufacturing capacity also takes time. Vaccines for clinical trials are generally made in small batches in pilot facilities that aren’t capable of producing commercial quantities. But because very few candidate vaccines make it through clinical trials successfully — Graham puts the number at less than 10 percent — manufacturers are understandably reluctant to invest in large-scale production facilities until they know the vaccine will work. This adds an additional time lag to the vaccine-development process.
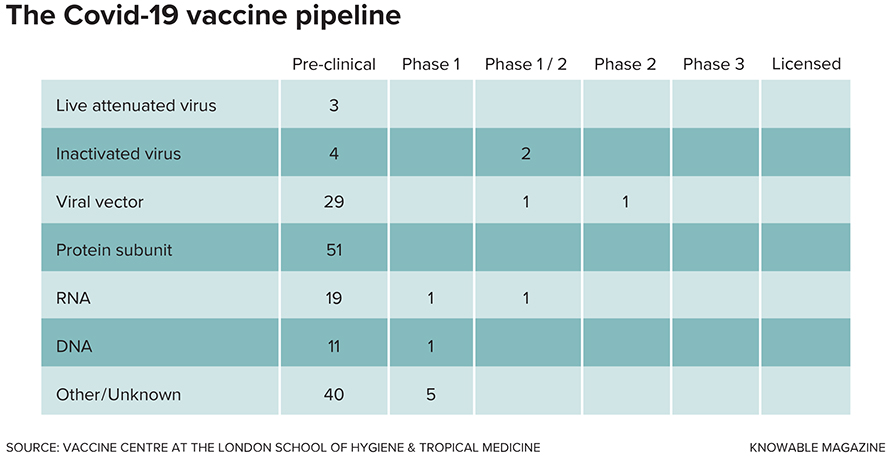
How can we speed up that process?
With COVID-19, scientists already have a big head start, because this isn’t the first coronavirus they’ve tried to make a vaccine for. They had begun making vaccines for SARS and MERS during their outbreaks in 2003 and 2012, respectively, only to abandon the efforts when the outbreaks receded.
So when COVID-19 came along, researchers already knew a good target for a vaccine: the spike protein that sits on the surface of the virus, and especially the part that binds to human cells, enabling the virus to gain entry. Researchers even knew how to stabilize that key part of the spike protein so it holds its shape during vaccine production.
This advance knowledge enabled the biotech company Moderna, in collaboration with the US government’s Vaccine Research Center, to decide on a vaccine candidate within three days of the COVID-19 genome being sequenced. That’s nearly a year quicker than it took to find a candidate for a SARS vaccine in 2003-04.
Ideally, a steady stream of vaccine candidates should be entering clinical trials, so that each new trial can learn from its predecessors. “If I’m coming behind, I can design my studies better so I don’t make the same mistakes,” says Maria Elena Bottazzi, a vaccinologist at Baylor College of Medicine in Houston and coauthor of an article about vaccines for developing countries in the Annual Review of Medicine.
Graham is hopeful that vaccine developers can also speed through the time-consuming, large-scale Phase 3 trials by riding the wave of new COVID-19 infections that is widely expected this fall. By testing the vaccine in locations where large outbreaks are already occurring, researchers should be able to tell more quickly whether it really works.
Once testing shows that a vaccine candidate is safe and effective, regulators are likely to expedite its approval. “Everyone recognizes that this is a crisis, including the regulatory authorities. In this case, the benefit of having a vaccine earlier is very high,” Graham says. But that should not mean cutting corners on safety testing, he adds. “We have to be cautious, even though we have to go fast. I think we can do those things together if we pay attention.”
What could go wrong in testing candidate vaccines?
It’s unwise to pin too much hope on any given vaccine, because most candidate vaccines — Graham puts the number at more than 90 percent — fail during their clinical trials, usually at early stages. That’s why it’s essential to have many potential vaccines to test. “You’ve got to try multiple shots on goal, and some of them will work,” Yeaman says.
One big reason why vaccines fail is that they lead to the wrong kind of immune response. “There’s a big difference between an immune response and a protective immune response,” Yeaman says. To be effective, a vaccine must do more than merely provoke the body to make antibodies. Those antibodies must also be able to neutralize the virus so it can no longer invade host cells. A good vaccine should also prompt the right sort of activity from the body’s T cells, the part of the immune system that’s responsible for orchestrating the body’s immune response to the virus. Vaccines that do these things well in lab animals often disappoint in human trials, and only testing can weed out these failures.
Sometimes, vaccines can even make a disease worse. Two different processes can cause this. In one, certain types of antibodies induced by the vaccine can help the virus more easily invade a host cell. They do so by attaching both to the virus and to a receptor for antibodies on the cell surface, serving as a bridge between the two.
In the other process, the vaccine primes the immune system too vigorously, so that an infection by the virus later on provokes an immune overreaction — a “cytokine storm” — that can prove lethal.
Both of these problems have been reported in the past with animal studies of coronavirus vaccines, including vaccines that were being developed for SARS and MERS. But there is as yet no indication that people would react in the same way. “I don’t think the risk is extremely high — not as much as the risk of not having a vaccine and having the kind of mortality we’re going to have if everyone becomes infected with this virus,” Graham says.
Is there a risk that we might never get a vaccine for COVID-19?
It’s possible, but unlikely. There are a few viruses out there that have stubbornly resisted all efforts to develop a vaccine, including hepatitis C, herpes simplex and HIV. But many of these viruses have special features that help the virus evade a vaccine. There is no indication that the virus causing COVID-19 has any such features, Yeaman says.
On the positive side, veterinary researchers have successfully developed vaccines for other coronaviruses that infect livestock. And earlier attempts to develop vaccines for SARS and MERS — both closely related to the COVID-19 virus — showed promising initial results before those diseases receded and the vaccine programs were abandoned. “We’re hopeful that this virus is going to be amenable to vaccine,” Graham says.
Indeed, in mid-May, Graham’s group, working with Moderna, reported that eight healthy volunteers who received their candidate COVID-19 RNA vaccine developed a protective antibody response. (Much testing remains to be done, of course. It is still unknown whether the antibody response actually prevents disease, and Moderna has yet to share its full results.) Also in May, other researchers reported a promising T-cell response in patients who had recovered from COVID-19. Taken together, these results suggest that a vaccine is likely to succeed, Yeaman says.
What if a COVID-19 vaccine gives only partial protection against the virus?
It’s still better than nothing. Some existing vaccines — flu is a good example — are useful even though vaccinated people still sometimes get sick, because they reduce the incidence of severe illness and death, Bottazzi says. It’s also possible that a partially effective vaccine, in combination with a partially effective antiviral drug, could add up to nearly full protection, Yeaman points out.
After we’ve manufactured the vaccine, are we home free?
Not yet. Even at the point when manufacturers are producing vast quantities of a vaccine, the job isn’t done. “A vaccine is not just going to magically appear in people’s homes,” says Bruce Y. Lee, a vaccine logistics expert at the City University of New York. “Coming up with a clear distribution and implementation plan is very important — and it’s challenging.”
Lee studies the supply chain for vaccines — that is, the intermediary steps needed to deliver vaccines from the manufacturer to the point of vaccination. This chain can involve many layers. During the 2009 influenza pandemic, for example, vaccine manufacturers shipped to central hubs, which then delivered to individual states, and those state governments distributed the doses more locally. The system was plagued by mismatches between supply and demand, with far too little vaccine early on, and too much later.
At every step, this distribution process requires people, space and often refrigeration, because many vaccines are unstable at room temperature. A sudden surge of hundreds of millions, or even billions, of COVID-19 vaccine doses is likely to overwhelm the system, especially in lower-income countries where adequate refrigeration is already an issue. “The existing supply chains are not ready for this,” Lee says.
Even if vaccine production ramps up gradually, the supply chain will need to ensure that the vaccine initially goes to those in greatest need, such as health care workers, the elderly and others at higher risk. Health officials may also want to integrate vaccination with testing, Lee suggests, so that scarce vaccines do not go to people who have already had COVID-19.
Even something as simple as the size of vials for the vaccine and their packaging can make a huge difference in ease of delivery. Packaging for a rotavirus vaccine distributed in the early 2000s, for example, was so bulky that it clogged supply chains in Latin America and slowed distribution of all vaccines until manufacturers reformulated to allow smaller packaging, Lee says.
The problem gets even harder if the COVID-19 vaccine, like some existing vaccines, turns out to require two doses. Not only would that double the number of doses to be shipped, but front-line workers would need to do careful tracking to ensure that each recipient got exactly two doses, with the proper interval between them.
These logistical issues need attention now, not when the vaccine is ready, Lee says. Indeed, supply chain requirements might even affect which candidate vaccines we choose to pursue. A single-dose, unrefrigerated vaccine, for example, would be much preferable to a two-dose vaccine with strict refrigeration needs. “This has to be looked at as a whole-system issue,” Lee says.
How can we be readier next time?
In a sense, the world caught a break with COVID-19. “We were lucky, in this case, that this was a coronavirus, because we sort of knew how to make an antigen,” Graham said in an online lecture in April.
We might not be so lucky next time — and there will be a next time.
To have the best chance of developing a vaccine quickly, experts should start now to develop at least one prototype vaccine for each virus family known to infect people, Graham says. (So far, that’s only been done for about half of the roughly two dozen families.) That way, whatever virus emerges next, vaccine developers will have a known starting point, as they did with the COVID-19 virus. “The more information you can have ahead of time, the better off you’re going to be in responding,” Graham says.
New vaccine technologies, such as RNA vaccines, would allow authorities to build vaccine factories that could quickly adapt to produce new vaccines, since the same production line could copy any RNA sequence, whereas producing proteins or whole viruses requires more bespoke production. This would eliminate the need for new construction. Such vaccines could also be made in smaller, more decentralized factories, which could ease supply-chain problems.
This time around, we probably won’t have a vaccine until next spring at the earliest, or perhaps the fall of 2021. Eighteen months may seem like a long time to wait, but it’s worth remembering that if scientists hit that optimistic target, they will have developed a vaccine far faster than it’s ever been done before.
This article originally appeared in Knowable Magazine, an independent journalistic endeavor from Annual Reviews.
Enjoy reading ASBMB Today?
Become a member to receive the print edition four times a year and the digital edition monthly.
Learn moreGet the latest from ASBMB Today
Enter your email address, and we’ll send you a weekly email with recent articles, interviews and more.
Latest in Science
Science highlights or most popular articles
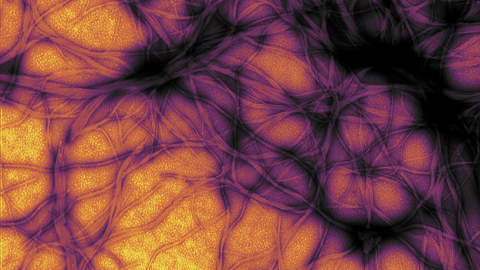
Before we’ve lost what we can’t rebuild: Hope for prion disease
Sonia Vallabh and Eric Minikel, a husband-and-wife team racing to cure prion disease, helped develop ION717, an antisense oligonucleotide treatment now in clinical trials. Their mission is personal — and just getting started.
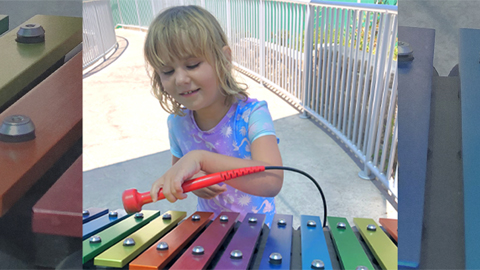
Defeating deletions and duplications
Promising therapeutics for chromosome 15 rare neurodevelopmental disorders, including Angelman syndrome, Dup15q syndrome and Prader–Willi syndrome.
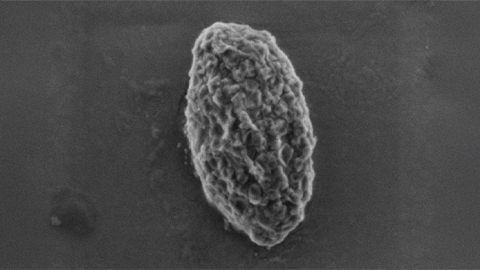
Using 'nature’s mistakes' as a window into Lafora disease
After years of heartbreak, Lafora disease families are fueling glycogen storage research breakthroughs, helping develop therapies that may treat not only Lafora but other related neurological disorders.
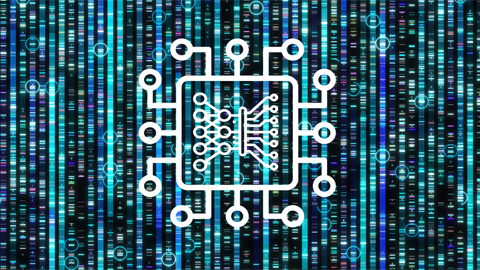
Cracking cancer’s code through functional connections
A machine learning–derived protein cofunction network is transforming how scientists understand and uncover relationships between proteins in cancer.
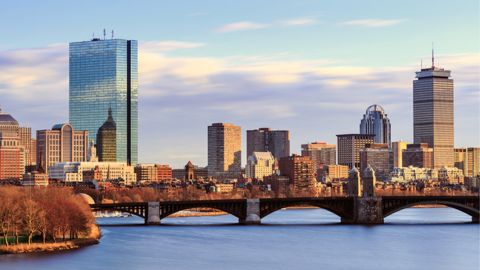
Gaze into the proteomics crystal ball
The 15th International Symposium on Proteomics in the Life Sciences symposium will be held August 17–21 in Cambridge, Massachusetts.
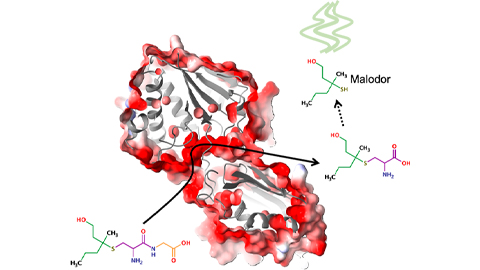
Bacterial enzyme catalyzes body odor compound formation
Researchers identify a skin-resident Staphylococcus hominis dipeptidase involved in creating sulfur-containing secretions. Read more about this recent Journal of Biological Chemistry paper.