Organizing fat: Mechanisms of creating and organizing cellular lipid stores
Life is unpredictable, so cells create nutrient reservoirs that enable them to subsist during prolonged starvation. Cells generate lipid droplets, or LDs, the primary nutrient reservoirs of eukaryotes. These lipid storage organelles, composed of a phospholipid monolayer surrounding a neutral lipid core, form on the surface of the endoplasmic reticulum, or ER, which generates almost all the lipids within LDs.
In many cells, LDs maintain contact with the ER for extended periods, so ER–LD interorganelle crosstalk is essential for cellular lipid homeostasis. We know that LDs play important roles in energy and cellular homeostasis, but recent studies also highlight noncanonical roles for LDs in lipotoxicity control, development, cell signaling and even reservoirs for hydrophobic metabolites.
LDs can remain in cells for extended periods before metabolic cues mobilize them. They often are mobilized in periods of energetic crisis, prompting the question: How do cells organize their LD stores to maximize storage and harvesting efficiency?
Uncontrolled LD mobilization can spill toxic fatty acids into the cell interior. Fatty acids act as biological detergents that can dissolve membranes, so cells must regulate LD production, storage and mobilization.
Studies have found that LD production and turnover are highly regulated, establishing the existence of spatial determinants that govern not only where LDs form within the ER network but also where they accumulate within the cytoplasm. Here, I briefly summarize some of these findings and how they reveal a remarkable spatial organization in lipid metabolism and LD biogenesis.
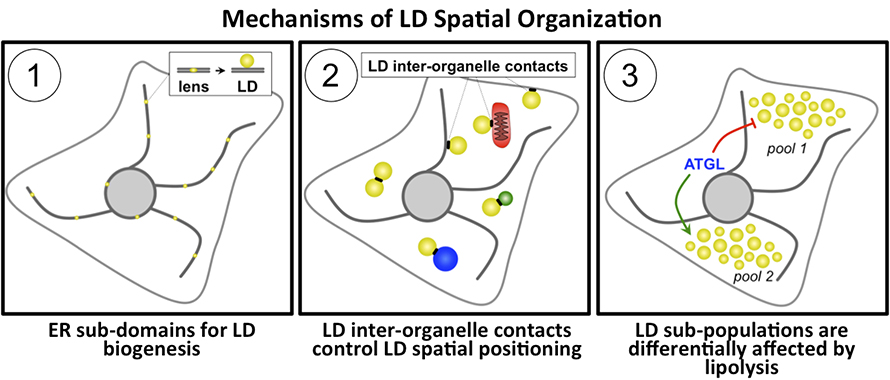
1. The ER network contains lipogenic hot spots that organize lipid processing reactions and LD biogenesis:
Many recent studies of LD formation and emergence from the ER network focus on seipin, an ER-resident protein linked to a severe form of congenital generalized lipodystrophy, or abnormal fat storage. Studies show that seipin accumulates at neutral lipid lenses within the ER network where seipin oligomers support the growth of nascent LDs. Researchers now view nascent LD lenses as lipogenic ER subdomains containing structural factors and enzymes that drive lipid synthesis reactions. In addition to seipin, our group has identified Snx14, a member of the sorting nexin protein family, as a tether that promotes LD biogenesis at ER–LD contact sites. Snx14 is highly conserved, and orthologs in yeast and Drosophila play similar roles in ER–LD crosstalk, underscoring the functional conservation of LD tethers across evolution.
2. Protein tethers connect LDs to other organelles, regulating LD positioning and use in the cell:
In addition to being tethered to the ER as they mature, LDs are connected to other organelles, and this provides an intuitive mechanism to arrange them spatially in the cell. Elegant studies have found proteins that enrich at interfaces between LDs and mitochondria, LD–peroxisomal contacts, LD–plasma membrane contacts, LD–lysosome contacts, and even contacts between LDs. Many of these studies show that LD interorganelle junctions are sites of lipid exchange between the LDs and recipient organelles.
3. LD mobilization is highly regulated, and cells appear to contain pools of LDs that are created and mobilized under specific nutrient cues:
During fasting or in response to cellular cues, cytoplasmic lipases mobilize LDs to liberate free fatty acids and glycerol into the cytoplasm. Hyperactive lipolysis can disrupt cellular homeostasis, so regulatory mechanisms fine-tune LD synthesis and breakdown. Some regulate the recruitment and activity of lipases on the LD surface, and others control the creation of LD subpopulations that are mobilized differentially by lipolysis.
Our lab uses Drosophila tissue to study how adipocytes control LD storage and mobilization. We have found that larval adipocytes contain spatially distinct LD subpopulations that respond differentially to LD mobilization. A pool of small LDs near the cell surface are mobilized when the organism fasts and multiply during high-nutrient feeding. This indicates that LD positioning in the cytoplasm may dictate lipolysis. Similar mechanisms are present in yeast and mammalian tissues. In yeast entering into stationary phase growth, LDs tend to grow and accumulate near the cell’s digestive vacuole, where some LDs are delivered to be broken down during low-nutrient subsistence. In mouse enterocytes, pools of LDs derived from lipid absorption at the basolateral side of the cell are mobilized by intestinal adipose triglyceride lipase, or ATGL, activity, but lipids absorbed on the enterocyte apical surface appear to resist ATGL lipolysis and are instead shunted into chylomicron synthesis for export. This all implies that distinct lipid pools are maintained within cells and are exposed selectively to lipolysis. It suggests that positioning of LDs and lipid pools determines lipid storage and mobilization.
Many questions remain. How are distinct lipid pools and LD subpopulations maintained and demarcated by the cell? How do cells decide where to create hot spots in the ER network where nascent LDs are created and emerge? Is LD biogenesis a protein-driven event, or are proteins mere facilitators of lipid-driven LD biogenesis?
We hope to address these and other questions as new tools and technologies emerge.
Enjoy reading ASBMB Today?
Become a member to receive the print edition four times a year and the digital edition monthly.
Learn moreGet the latest from ASBMB Today
Enter your email address, and we’ll send you a weekly email with recent articles, interviews and more.
Latest in Science
Science highlights or most popular articles
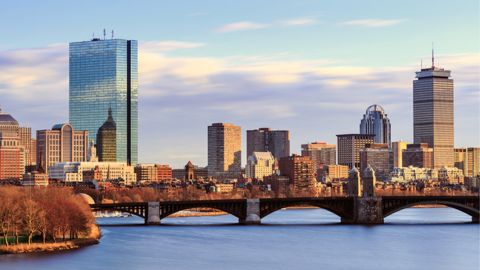
Gaze into the proteomics crystal ball
The 15th International Symposium on Proteomics in the Life Sciences symposium will be held August 17–21 in Cambridge, Massachusetts.
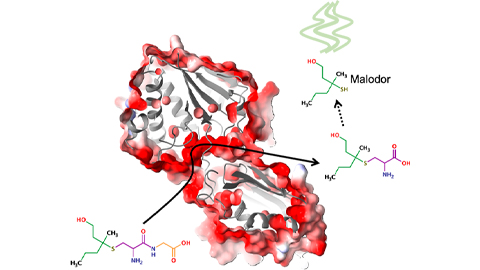
Bacterial enzyme catalyzes body odor compound formation
Researchers identify a skin-resident Staphylococcus hominis dipeptidase involved in creating sulfur-containing secretions. Read more about this recent Journal of Biological Chemistry paper.
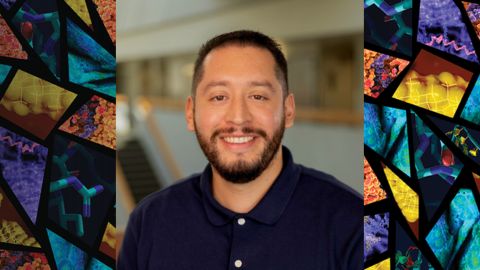
Neurobiology of stress and substance use
MOSAIC scholar and proud Latino, Bryan Cruz of Scripps Research Institute studies the neurochemical origins of PTSD-related alcohol use using a multidisciplinary approach.
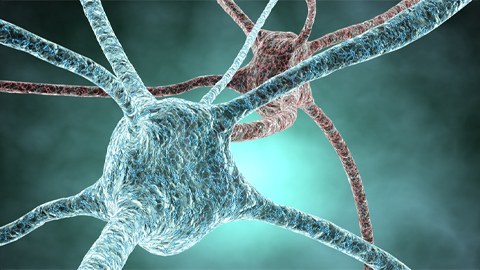
Pesticide disrupts neuronal potentiation
New research reveals how deltamethrin may disrupt brain development by altering the protein cargo of brain-derived extracellular vesicles. Read more about this recent Molecular & Cellular Proteomics article.
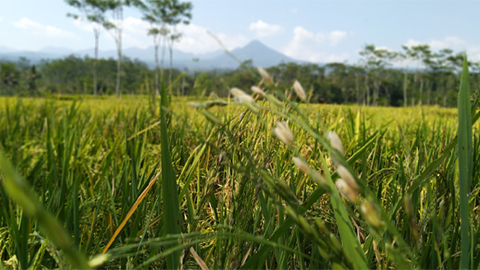
A look into the rice glycoproteome
Researchers mapped posttranslational modifications in Oryza sativa, revealing hundreds of alterations tied to key plant processes. Read more about this recent Molecular & Cellular Proteomics paper.
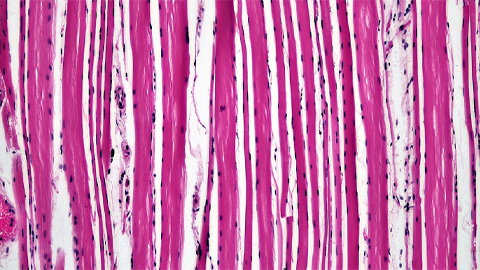
Proteomic variation in heart tissues
By tracking protein changes in stem cell–derived heart cells, researchers from Cedars-Sinai uncovered surprising diversity — including a potential new cell type — that could reshape how we study and treat heart disease.