A zest for synthetic biology
Orange zest wouldn’t be quite so zesty without limonene. And home bakers and mixologists aren’t the only ones who appreciate it. Limonene, which constitutes all but 5% of the essential oil of orange peels, is used industrially as a source of flavor for foods and of scent for cosmetics and household products. It also is used as a starting point for various pharmaceutical products and as a solvent and degreasing cleaner.
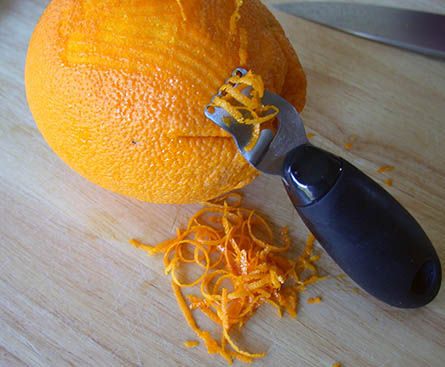
The limonene used in these applications is a byproduct of the juice industry. The Soudijin family of Bradenton, Florida, trades in limonene and about a dozen other citrus byproducts at their company, Florida Worldwide Citrus. According to product manager Matthew Soudijin, a grandson of the company’s founder, the price of limonene has spiked around the world during the COVID-19 pandemic as surges in demand for cleaning products crash into delays in citrus harvests and processing caused by global shutdowns and slowdowns.
Business was already tough. Florida’s orange harvest has plummeted in recent years as citrus greening, a bacterial infection spread by insect pests, spreads across the state. While Florida Worldwide Citrus once dealt mainly in local products, most of its supply now comes from growers in other countries. Unpredictable weather, on the rise worldwide, can damage crop yields further.
“The price tends to fluctuate with the harvest,” Soudijin said, adding that these variations can make for unhappy customers. “If beverage and flavor companies are paying $2 a kilo, and then twice as much six months later, they see that as a problem.”
To stabilize their production costs, those companies would prefer to use a reliable synthetic source. But although limonene is a simple molecule, it has a chiral hitch: a carbon whose bonds can be arranged in two ways, producing either D- or L-enantiomers. Whereas citrus fruits produce only D-limonene, many synthesis methods produce a mixture of the two enantiomers. The mixture, called dipentene, works well enough for degreasing oil shafts, but it can’t be used for fragrance and flavor purposes, because while D-limonene has a pleasant citrus odor, L-limonene smells like turpentine.
This is exactly the kind of problem that synthetic biologists like to solve using enzymes, which are stereoselective by nature. Industrial production of vitamins, penicillin, amino acids and many other chiral products depends on commercial-scale fermentation using microbes engineered to conduct molecular transformations that turn sugar from the media into more valuable molecules. But unlike many of those products, limonene is toxic to cells.
Sarah Reisinger, vice president for research and development in biotechnology at the flavor house Firmenich, told Perfumer & Flavorist magazine in April, “I believe, in the coming years, the pace of renewable biotech ingredient introduction will increase.” She added, “I believe that biotech products, in addition to renewability, offer the reliability, consistency of product composition and cost that the industry needs.”
Historically, limonene has been available so cheaply that overcoming challenges to producing it through fermentation wasn’t worth the investment. But now, with a $300 million market at risk, could limonene become one of those biotech ingredients?
From tricks of the fermentation trade to entirely new approaches, researchers in academia and industry have developed a variety of ways to keep their organisms alive while also optimizing expression and production of limonene — and some new approaches whose proponents argue that “alive” is overvalued. They’re getting better at making limonene and related molecules more efficiently. Whether these approaches will yield enough efficiency to bring biofermented limonene to market remains to be seen.
Working toward monoterpenes
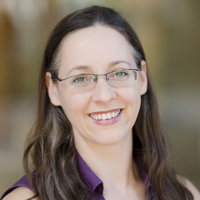
Modifying an organism to produce a novel molecule can be a challenge. New metabolic pathways may steal energy or building blocks from other processes that the cell needs to stay alive, putting stress on the organism. In some cases, at high enough levels, the desired product or its metabolites may even prove toxic to the organism.
Claudia Vickers is director of the Future Science Platform in Synthetic Biology at Australia’s Commonwealth and Industrial Research Organisation and also has a lab at the University of Queensland. She focuses her academic work on engineering microbes to generate monoterpenes, a class of molecules including limonene that are unusually difficult to produce.
“What’s interesting about (terpenes) is that they’re modular,” Vickers said. “They start with a five carbon product that has a prenylphosphate moiety, and there’s a series of condensation reactions to make molecules with five, 10, 15, 20 (carbons).” After this concatenation of subunits, other enzymes use energy from the remaining phosphate group to make molecular transformations that diversify the terpenoid family: twisting the molecule into cyclic structures or oxidizing its double bonds to add methyl or hydroxyl groups.
Some 70,000 terpenes and terpenoids are known. Their functions are nearly as numerous. Plants use terpenes for defense against herbivores and as components of resins, hormones and cell membranes; the smallest, like limonene, are volatile and involved in intraspecific and cross-species recognition. Vickers, who trained as a plant biologist, said, “They’re involved in almost everything.”
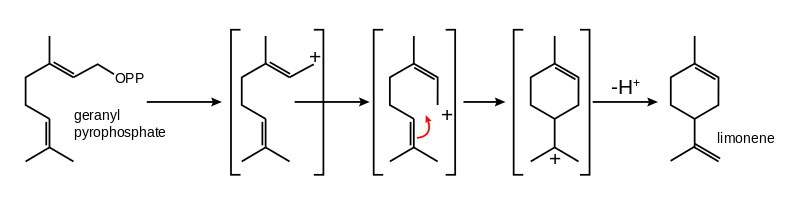
All cells generate isopentenyl pyrophosphate and dimethyl pyrophosphate, the two five-carbon precursors, and most use them to make much larger lipids like sterols. But plants, somewhat unusually, are able to generate the 10-carbon molecules known as monoterpenes, such as menthol, limonene and pinene. Cajoling microbes to produce these molecules is a challenge, Vickers said.
The Future Science Platform keeps a catalog of DNA coding for enzymes with various functions from various organisms. Vickers calls it a parts repository, conjuring a hybrid between Addgene and AutoZone. Limonene is a popular target terpene among metabolic engineers because there is a part, an active and well-characterized enzyme, that makes it in a single step from a 10-carbon precursor called GPP.
In principle, it should be possible to express that enzyme in an organism that already makes GPP and then harvest the product. But Vickers cautions against such simplistic thinking.
“It’s important to understand, you can’t go in, massively upregulate everything, brush your hands and go, ‘Right, job done,’” she said. “That often doesn’t give you the product. It’s often all about balancing flux in the pathway.”
For example, in brewer’s yeast, the enzyme that joins IPP and DMAPP subunits also immediately adds a second IPP subunit. Feeding in more precursors in hopes of boosting GPP production without modifying that enzyme would not increase the amount of limonene in the cell; it simply would result in more of the 15-carbon product. But removing the offending enzyme outright is not an option, because the cell requires metabolites later in its synthetic pathway to stay alive.
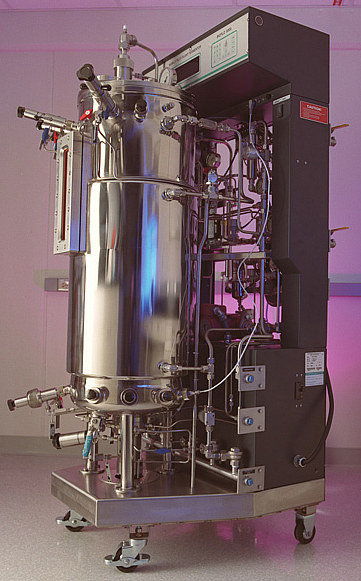
and harvest their products.
Vickers credits a postdoc in her lab with devising a solution involving conditional degradation of the enzyme that competes against limonene synthase. By combining inducible degradation with sensing of the required product, Vickers said, “We got a balance between healthy growth of the cells and channeling carbon off to the monoterpene.”
Trial-and-error approaches like this are staples of metabolic engineering. Scientists introduce a change and then use metabolomics to assess its effect on an engineered microbe and identify any bottlenecks upstream of the desired product.
Should someone find a way to produce high titers of limonene without cannibalizing another essential metabolite, there’s another problem waiting: At a high enough concentration, limonene and other monoterpenes can kill microbes that produce them. The small, hydrophobic hydrocarbons are thought to incorporate into cell membranes and compromise the integrity of the cell.
“To date, most of the production of these compounds has not reached levels that are high enough to actually produce toxicity,” Vickers said, “which is unfortunate, because we’re trying to get to high levels!”
Addressing toxicity
Synthetic biologists have found ways to remediate the harmful effects of natural products they wish to produce in bulk. After all, even ethanol kills microbes at a high enough concentration; that has not stopped humans from producing it by fermentation for millennia.
Jay Keasling, a professor at the University of California, Berkeley, who founded the synthetic biology company Amyris, said, “I wouldn’t say that they’re all easy, but there are some tricks.”
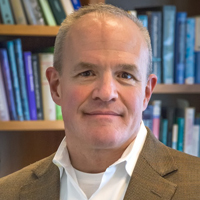
in a lab at the Joint Bioenergy Institute.
The most popular trick is to choose a new microbe, one with higher tolerance for the product. That works best if an easily engineered alternative organism tolerates the molecule better. If not, some labs use directed evolution, growing the microbe of interest in increasing concentrations of the desired product to select for mutations that yield more resilience.
Engineers also can take steps to mitigate the harmful compound’s effects on the microbe: for instance, incorporating transporters to remove it from the cell, designing the pathway to sequester the product in an organelle that will keep the harmful molecule away from important cellular functions, or adding a modification such as a sugar that renders the molecule nontoxic and then is removed after isolating the product. Keasling’s lab once achieved a 90-fold increase in limonene yield by outfitting E. coli with a metabolic pathway to boost production of a precursor molecule and a cytochrome that hydroxylated the limonene, preventing it from integrating into membranes.
There are also solutions completely external to the cell. According to Vickers, the most effective way to diminish the harmful effects of limonene is to grow the organisms producing it in an emulsion of a nontoxic organic solvent, which extracts the hydrophobic limonene. “The moral from that story is that we often, as metabolic engineers, try to look for fancy genetic solutions,” she said. “But there might be a much simpler process solution.”
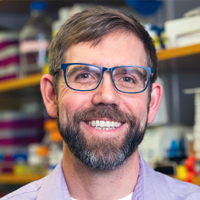
Beyond rendering a new metabolic pathway survivable, some biologists say, there is a more fundamental conflict at the center of metabolic engineering. Michael Jewett, a professor and director of the Center for Synthetic Biology at Northwestern University, said that cellular systems’ tendency toward homeostasis often clashes with the functions an engineer would like to introduce.
“I want to make a whole lot of something the cell doesn’t care about,” Jewett said. “So we end up in this tug of war … between what the cell’s evolutionary and adaptation objectives may have led it toward and my engineering process objectives, which are titers, rates and yields.”
Jewett’s lab is one of a few that are working on an unconventional approach to resolve the conflict. “We just cut the rope,” he said. “Rather than focus on the chassis organism itself, (we) focus on the molecular machinery — not only within the organism but outside of it.”
Synthetic biochemistry
Jewett’s lab often works with cell-free production systems made from bacterial lysates, which he says offer some convenient features of a biological system but do not force the engineer to fight as hard against the cell.
“Cell-free systems … can produce molecules that are otherwise toxic to cells,” he said. “They can actually use toxic feedstocks, like pretreated biomass; and we also look at it as a really exciting prototyping strategy.”
By carrying out cell-free translation of enzymes in lysate from E. coli and then mixing the enzyme-enriched lysates in a process he compares to mixing a cocktail, Jewett’s lab dodges the hurdle of tweaking the expression levels of numerous enzymes introduced into a genome.
“You can reduce the problem of synthesizing biosynthetic pathways to one of simply mixing solutions by liquid handling,” he said.
The method keeps many of the beneficial aspects of a cell system; for example, in raw lysate, glycolysis constantly produces energy and enzyme cofactors from sugars. It eliminates any problems that may arise from a toxic product. In a recent paper on limonene biosynthesis, Jewett’s lab reports on cell-free optimization of a synthetic pathway that converts glucose into limonene in nine enzymatic steps. By testing the efficiency of homologous enzymes from different organisms, they found that certain combinations unexpectedly produced dramatic improvements in yield.
“Frankly, we’re limited only by the ability to analyze the samples,” Jewett said. “We could make 100,000 different combinations of pathway enzymes, but that would take my grad student a long time with the (mass spectrometer).”
The research team regards their approach as a tool for prototyping in advance of engineering new strains; once the highest-yield pathway is found, they say, it can be introduced into a microbe.
“Activities in a pot”
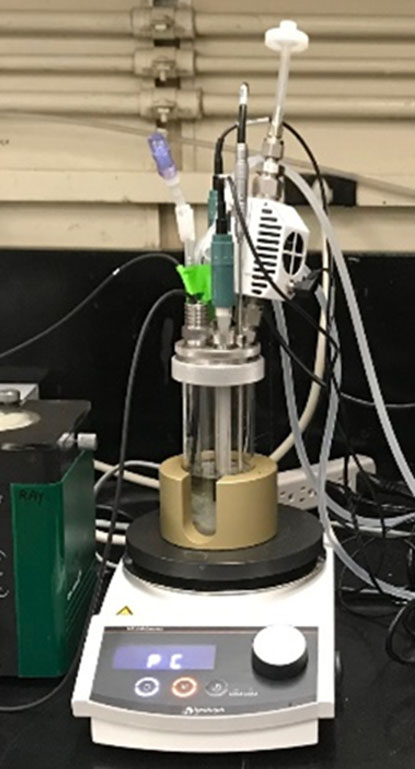
Even farther from a cell system is the synthetic biochemistry approach Jim Bowie, a professor at the University of California, Los Angeles, is pioneering in the lab and at the company he co-founded, Invizyne.
“The idea is very simple,” Bowie said. “We have a biochemical transformation that we want to perform, and a series of enzymes that carries out those steps. So let’s just express those enzymes … and put those activities in a pot. And now we have a biochemical pathway.”
This approach relies heavily on purification; the laboratory introduces only the enzymes and cofactors directly involved in conversion of glucose to a desired product into a stripped-down reaction mixture. Bowie argues that this approach is even easier than a system of lysates to tweak and optimize; it produces a simpler mixture of products, which makes for easier purification down the line. As in Jewett’s method, toxicity is no object.
Of course, enzymes consume cofactors such as ATP and NADH in the course of their activity. Supplying a steady stream would be costly; this is one of the useful functions cellular homeostasis provides when it’s present.
“The trick is cofactor recycling,” Bowie said. But he doesn’t hope for a system with perfect stoichiometric balance between cofactors added and consumed; setting up a system like that, he said, is “basically asking for a perpetual motion machine.”
Instead, Bowie’s lab devised what he calls a molecular rheostat for ATP that involves two competing glycolysis pathways that depend on the concentration of phosphate in the system. An enzyme borrowed from the Krebs cycle converts glyceraldehyde-3-phosphate into 3-phosphoglyceric acid, replenishing ATP. A second enzyme performs the same conversion but without generating ATP and tends to outpace the first enzyme when ATP levels are high and free phosphate is low. Together, the two competing reactions balance ATP level. Bowie calls a system designed on similar principles for NAD regulation a purge valve.
The approach adds a few layers of complexity back to a simplified system and costs more up front than relying on live microbes, because all the enzymes must be harvested and purified. Bowie argues that, with sufficiently stable engineered enzymes, the increased cost should amortize over time. Still, his approach has its detractors.
“I think that most people don’t think it’s realistic to put these complex systems together with free enzymes,” Bowie said. But he’s optimistic. “We already know that metabolic engineering is extremely challenging. We already know that. Why not give this approach a chance?”
“Frankly, it works surprisingly well,” Jewett, who was not involved in the work, said about the molecular purge-valve system. Invizyne has landed small business innovation research funding and enjoyed some preliminary interest from flavor and fragrance companies, although they’re more interested in the commercial possibilities of synthetic cannabinoids, which may command a higher price.
Mechanismless optimization
Synthetic biochemistry involves tight control and design of a pathway; every component is planned and adjusted. Biologists, computer scientists and chemists are taking a radically different approach to optimizing biosynthetic pathways at the company Zymergen.
As flavor and fragrance companies contemplate producing limonene through biofermentation and academics explore how to do that, veterans in the synthetic biology industry are hesitant to jump aboard. Their reasons come down to economics and past experience.
Zach Serber, co-founder and chief scientific officer of Zymergen, said, “If you have a microbe making a drop, a tiny amount of something interesting, that’s tantalizing … but wildly insufficient for commercial production. You need to be making truckloads, train car loads of the stuff, efficiently.”
Engineers cannot always reach that efficiency by tweaking the known components of a system, Serber said.
“You often fall short of your commercial goals. You reach a wall, whereby optimizing the known components of biology doesn’t seem to be sufficient,” he said. Serber argues that the challenge stems from biology’s many unknowns.
“The vision of making biology like electrical engineering may come true. But it certainly ain’t easy and has been slow in coming,” he said. “Because unlike capacitors and resistors … the parts available to do biology have evolved; we don’t usually know what they do, and they interact with one another in peculiar, unexpected ways.”
At Zymergen, Serber said, instead of using analytical chemistry to hunt systematically for bottlenecks in a metabolic pathway, “We treat the genome of a microbe as an optimization landscape, a design space, where the phenotype you’re trying to improve — the efficient production of a given biomolecule — is going to be affected by all the genes in the genome, in ways you can’t necessarily predict.”
Outlook
Will limonene ever be made in commercial quantities by biofermentation? The answer depends not just on synthetic biologists’ success in resolving technical problems but also on producers outside the lab, their output and pricing decisions, and whether demand for the molecule continues to grow.
Limonene is just one of thousands of natural products that may be at risk in a changing climate. And looking ahead, synthetic biologists envision producing chemicals that never have been observed before in a cell or carrying out blends of organic and inorganic reactions using enzymes.
Back in Florida, the Soudijin family of Florida Citrus Worldwide has adapted to the changing market. In addition to buying fruit from around the world to make up for shortfalls in locally harvested product, they’ve invested in a more elaborate flavor extraction and concentration lab to produce flavors themselves. Among other offerings, they sell extracts and essential oils to companies that want to adjust the flavor of their orange juice but still use only orange-derived ingredients.
Still, the Soudijins are looking warily at the next few years, wondering what the future will bring for their industry.
Lessons from jet fuel
There was a time when synthetic biologists aimed to make biofuels, cheap ethanol and other products that could be burned as greener alternatives to petroleum-based fuel.
Back when Zach Serber was the director of biology at Amyris, the company focused on producing jet fuel wholly derived from sugarcane through fermentation. Though the company succeeded and gained regulatory approval for its fuel, the year that the greener fuel came to market, the price of crude oil dropped by almost half, making it impossible for Amyris to turn a profit on its product. The company dropped the product and survived by refocusing on more expensive chemicals, but the experience has scarred the industry.
According to Serber, scientists in the biofuels industry “went tilting at windmills. We went head to head against petroleum, trying to come up with transportation fuel based on biology and found that, especially when the price of oil collapsed, it couldn’t compete on price.”
Now, much of the industry regards the attempt to produce biofuels as a cautionary tale for anyone aiming at large-scale production of relatively inexpensive commodity chemicals.
“There’s no way you’re going to get a venture capitalist to put money into a biofuel at this point,” Bowie said. “A ton of people lost their shirts.”
Enjoy reading ASBMB Today?
Become a member to receive the print edition four times a year and the digital edition monthly.
Learn moreGet the latest from ASBMB Today
Enter your email address, and we’ll send you a weekly email with recent articles, interviews and more.
Latest in Science
Science highlights or most popular articles
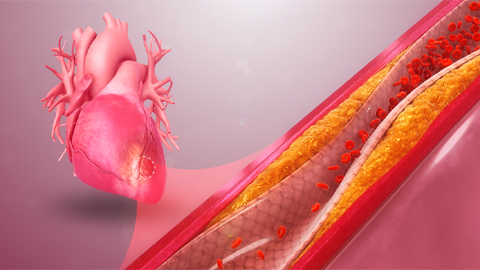
Scientists find unexpected correlation between age and HDL-C levels
In a 30-year multicenter study, researchers determined what factors predict HDL-C concentration. In their analysis, they found that HDL-C levels grew with increasing age and physical activity.
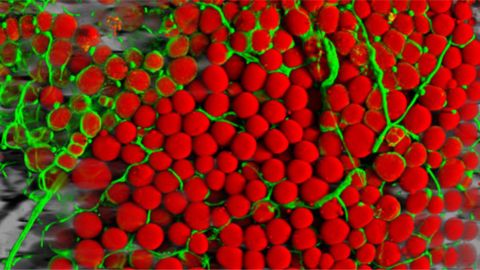
Butter, olive oil, coconut oil — what to choose?
Depending on the chain length and origin of the fat, regular fat consumption changes the specific makeup of fats in bloodstream and affect mild to severe cholesterol patterns. Read about this recent Journal of Lipid Research study.
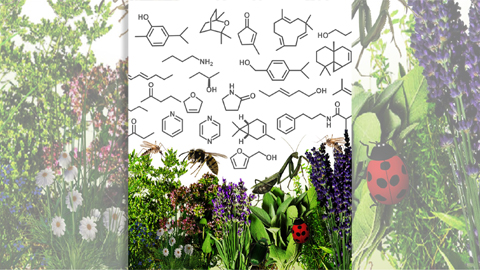
Computational tool helps scientists create novel bug sprays
Rapid discovery of mosquito repellent compounds is enabled through a novel screening platform that combines both computational modeling and functional screening.
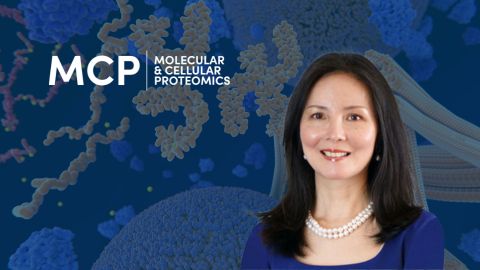
Meet Lan Huang
Molecular & Cellular Proteomics associate editor uses crosslinking mass spec to study protein–protein interactions to find novel therapeutics.
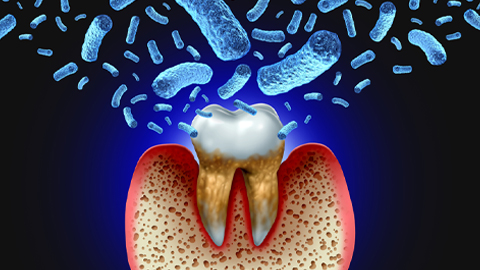
Influenza gets help from gum disease bacteria
Scientists discover that a protease from Porphyromonas gingivalis enhances viral spread. Read more about this recent Journal of Biological Chemistry paper.
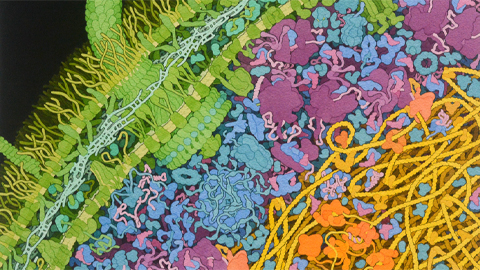
How bacteria fight back against promising antimicrobial peptide
Researchers find a mutation in E. coli that reduces its susceptibility to a potential novel antibiotic. Read more about this recent Journal of Biological Chemistry paper.