Taking optical microscopy by STORM
Xiaowei Zhuang at Harvard University is one of the pioneers in the field of super-resolution microscopy, which overcomes the problem of the diffraction limit in optical microscopy. First described in 1873 by one of the founders of optics, Ernst Abbe, the diffraction limit prevents researchers from using light to distinguish two objects that are apart by 200 nanometers or less. The field of super-resolution microscopy was recognized by the 2014 Nobel prize in chemistry.
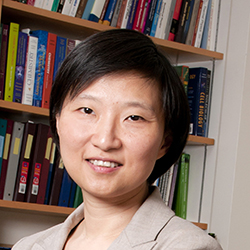
In 2006, Zhuang’s team was among the first to describe a method to overcome the diffraction limit. The method is called stochastic optical reconstruction microscopy, better known as STORM.
In STORM, a weak ray of light is used to stochastically turn on a small subset of photoswitchable probes. After the first subset of probes is imaged and localized, it is turned off. Then a different subset of probes is turned on, imaged and turned off. The process is repeated thousands to tens of thousands of times. The final high-resolution image is a reconstruction of molecular positions determined from the thousands of collected images.
Zhuang, a Howard Hughes Medical Institute investigator, and her team are pushing the boundaries of super-resolution microscopy and applying it to biological problems. The group is also working on transcriptome imaging based on single-molecule fluorescence microscopy.
What’s the cutting edge with STORM?
In terms of technology development, there are a number of things that are very important. One still is the spatial resolution. The first-generation work of STORM got resolution that’s 10 times better the diffraction-limited resolution. That 10-times-better resolution allows you to see a lot more inside biological specimens than we could see before. But if you could get to approximately 1-nanometer resolution, then you (could) open up another new window. That’s not incremental. It would allow you to study a lot of important problems that we still cannot do with super-resolution imaging.
There’s also the dynamics side. There, two aspects are important: the time resolution and the amount of dynamic information. The simplest way to understand the second aspect is if you have (to think about) a movie. A continuous movie of 100 frames can give a very good idea of the real-time process. If you only get five frames, you get a very choppy movie. With super-resolution imaging, whichever method you use, the number of frames one can get often is limited.
I can tell you about some of our applications. One area is in neuroscience. We discovered a membrane skeleton structure in the neuron using STORM. It’s a beautiful structure in the axons. We saw these highly ordered periodic rings of actin that are connected by spectrin tetramers. This structure is important for the mechanical properties and functions of axons. And it anchors many important membrane molecules and enzymes, so there might be functions in addition to (the) mechanical functions. Mutations in some of its molecular components are found in diseases, so we’re also studying its disease relevance.
(Another area is) how DNA is structured in the nucleus. We know a huge amount of one-dimensional information of the DNA, such as the DNA sequence, the modification profile and the protein-binding profile of the chromatin. But there is accumulating evidence that the three-dimensional structure of chromatin and the dynamics (of the structure) are also very important for the regulation of gene expression, replication and other functions. We’re using STORM to learn what the 3-D structure of chromatin and chromosome is like.
What are you doing with transcriptome imaging?
The goal is to get the spatially resolved transcriptome of individual cells. For many different types of cells, RNAs are not uniformly distributed. The local distribution of transcripts is important for the building and maintenance of local structure. Different types of cells have different gene-expression profiles, and that is important for cell fates, behaviors and functions.
There are about 60,000 different coding and noncoding RNAs (in a human cell). For each RNA, I could have oligo probes, each attached to different colored fluorophores. If I (were to) have 60,000 colors and if I could distinguish 60,000 colors simultaneously, I would be able to do transcriptome imaging. Obviously that’s not possible.
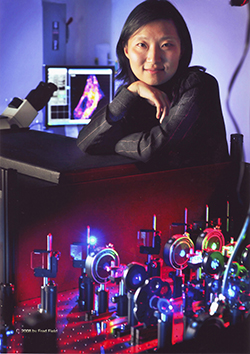
The other extreme is to use one color but image one RNA species at a time by flowing in (complementary) oligos (one set at a time). I can do it 60,000 times, provided that I have enough students and postdocs with enough patience and the cell is not damaged during that process! That’s equally unfeasible.
I came up with an idea that doesn’t require 60,000 fluorophores and doesn’t require 60,000 rounds of imaging: Image a combination of RNAs in each round but different combinations in different rounds. For example, we can encode RNAs with a binary code. Each RNA is associated with a code of 11011 and so on. In the first round, we only image those RNAs (whose) binary codes read 1 in their first digit. Then we quench those fluorophores and image a second set of RNAs whose binary codes read 1 in their second digit. In order to distinguish 60,000 different binary codes, I only need 16 rounds of imaging, because two to the 16th power is greater than 60,000. It makes something almost impossible, all of a sudden, possible.
However, I must say that this is an overly simplified picture, because identification of each bit has an error; accumulating errors from 16 rounds of imaging is severe. We solved the problem by using encoding schemes that can detect and correct errors.
How did you become interested in microscopy?
My Ph.D. thesis was on nonlinear optics, which is a spectroscopy approach. It had nothing to do with biology, but it gave me a strong training in optics. My supervisor was one of the pioneers in nonlinear optics, Ron Shen (at University of California, Berkeley). I used it as a tool to study liquid crystals, polymers and so on.
My first microscopy experience was when I was a postdoc at Steven Chu’s lab at Stanford. (Author’s note: Chu is a former secretary of energy for the Obama administration.) My work was looking at how RNAs folded. It was purified RNA molecules scattered on coverslips, and I inferred the conformation of RNA using a spectroscopy approach called FRET. I began to image biological specimens and study biomolecules without taking them out of the cell when I started my independent faculty position at Harvard.
What sparked your interest in science?
Probably family influence, because both of my parents are professors at the University of Science and Technology in China. I’ve always wanted to be a professor – even before I knew exactly what a professor was! I never changed my mind.
I knew that I was probably good at physics when I was little, because my dad told me that I had very good physics intuition. I always liked physics. Even into college, I did not quite like biology and did not think it was as elegant (as physics). Now I really love biology. The fact that there are so many unknowns makes it an extremely fascinating area to study.
What advice would you give to graduate students?
Be fearless. Also, it’s important to be very strongly motivated and willing to spend a lot of effort. Try not to get tunnel vision, but be open-minded to broadly learning about all kinds of new scientific frontiers. Good surprises await if you have an open mind, are fearless and are persistent when you encounter difficulties.
Watch Zhuang's plenary lecture from the ASBMB Annual Meeting:
Enjoy reading ASBMB Today?
Become a member to receive the print edition four times a year and the digital edition monthly.
Learn moreGet the latest from ASBMB Today
Enter your email address, and we’ll send you a weekly email with recent articles, interviews and more.
Latest in People
People highlights or most popular articles
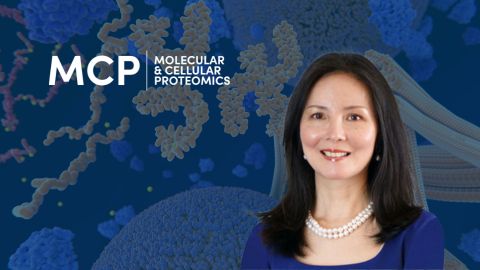
Meet Lan Huang
Molecular & Cellular Proteomics associate editor uses crosslinking mass spec to study protein–protein interactions to find novel therapeutics.
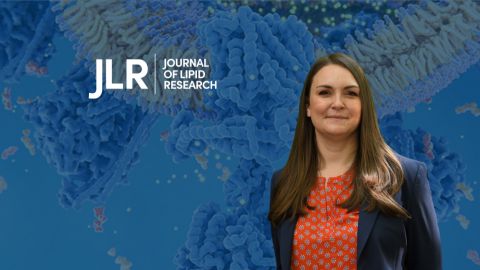
Meet Shannon Reilly
The JLR junior associate editor discusses the role of adipocytes in obesity at Weill Cornell Medical School.
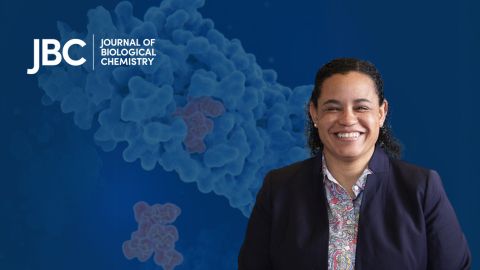
Meet Donita Brady
Donita Brady is an associate professor of cancer biology and an associate editor of the Journal of Biological Chemistry, who studies metalloallostery in cancer.
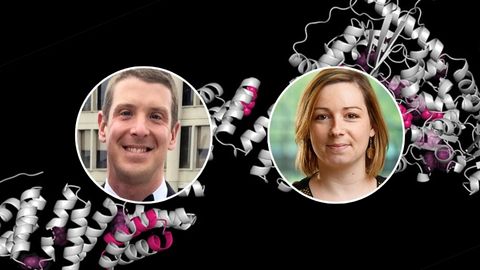
Glyco get-together exploring health and disease
Meet the co-chairs of the 2025 ASBMB meeting on O-GlcNAcylation to be held July 10–13, 2025, in Durham, North Carolina. Learn about the latest in the field and meet families affected by diseases associated with this pathway.
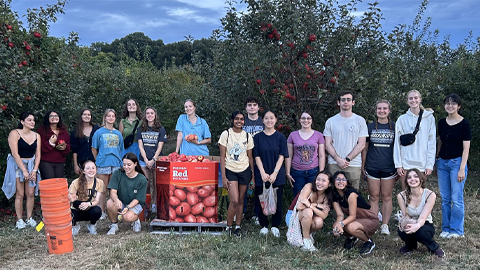
ASBMB recognizes 2025 outstanding student chapter
The Purdue group, led by Orla Hart, developed STEM outreach initiatives for low-income and minority students in Lafayette, Indiana.
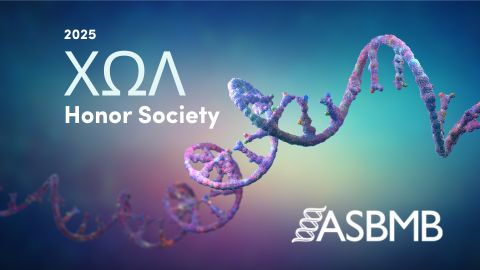
ASBMB inducts 2025 honor society members
Chi Omega Lambda, which recognizes exceptional juniors and seniors pursuing degrees in the molecular life sciences, has 16 new inductees in 2025.