Sports doping:
an extreme game of biology
As London gears up for the Summer Olympic Games, cheating athletes and antidoping officials continue their game of hide-and-seek. Doping is as old as sports itself, but the past few decades have seen the phenomenon grow more sophisticated. As our understanding of molecular biology, biochemistry, pharmacology and medicine improves, athletes become even more cunning in their exploitation of advances in these fields.
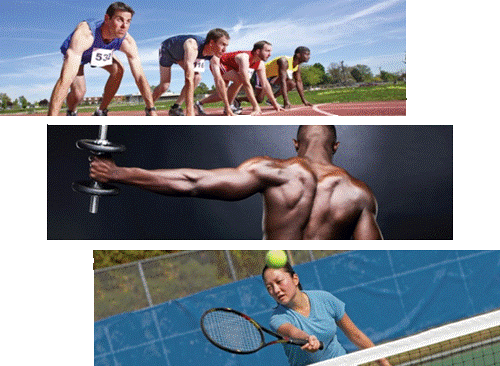
Enhancing sporting prowess goes back to the ancient Greeks, who used special diets and concoctions to improve their athletic abilities. In the 19th century, cyclists and other endurance athletes dabbled in molecules like strychnine, caffeine and cocaine. But doping exploded in the 20th century with advances in molecular biology and pharmacology. The Danish cyclist Knud Enemark Jensen died during competition at the 1960 Rome Olympic Games after taking amphetamines. With the introduction of synthetic anabolic steroids for increasing muscle mass in the 1960s, sporting authorities knew they had to take action. Testing for stimulants began in 1967; in the 1970s, the International Olympics Committee started to test for anabolic steroids.
These days, testing for performance-enhancing substances and techniques is routine and has a unified front. The World Anti-Doping Agency, established in 1999, is an independent foundation of the IOC. It works with intergovernmental organizations, governments, public authorities and other public and private entities to stay at the forefront of the fight against sports doping. WADA has research programs to support investigations into ways molecular biology, biochemistry, analytical chemistry and pharmacology can be applied to fight doping. It also maintains an extensive list of prohibited substances and methods for performance enhancement.
Abuse of biological molecules and drugs
Medicines, designer drugs and biological molecules are popular in the doping world despite the fact that authorities have safeguards in place for most of them. A classic example is erythropoietin, known as EPO. It’s a glycosylated protein hormone that stimulates bone marrow to produce red blood cell precursors. In the 1980s, the biotechnology company Amgen introduced the first synthetic version of EPO, called Epogen (1). The drug was designed to treat anemia in patients suffering from chronic kidney disease and other illnesses that cause a drop in red blood cell count.
But cheating athletes immediately saw the drug’s potential to increase their blood’s oxygen-carrying capacity during competition. Twenty-six years after the introduction of Epogen, synthetic variants of EPO still dominate the list of preferred doping agents. “EPO continues to be a problem because it’s so potent and works so well,” says sports doping expert Don Catlin at Anti-Doping Research. So many cyclists were caught doping with EPO and other drugs during the 1998 Tour de France that the media dubbed that year’s competition the “Tour de Shame.”
A detection method for EPO based on isoelectric focusing exists (2). But as Catlin notes, “There is a variety of ways to hide [EPO] and stay underneath the radar.” The method looks for the differences in glycosylation that make endogenous and synthetic versions of EPO migrate slightly differently on the gel. The biggest limitation of the test is that it can detect a synthetic EPO only if the drug is taken within two to five days of testing. The testing is also time and labor intensive, for it takes 48 hours to complete and is finicky. “In that context, it’s not the greatest test in the world,” says Catlin. “But it keeps nailing a small percent of those who are using EPO.”
Testosterone, an anabolic steroid, is another popular drug for abuse. Catlin’s group developed a carbon isotope ratio mass-spectrometric analytical approach in the 1990s to catch it (see sidebar). The test snagged U.S. sprinter Justin Gatlin, who won gold medals in the 2004 Games, and 2006 Tour de France winner Floyd Landis. Major League Baseball player Ryan Braun was accused of testosterone doping earlier this year but had his positive test result overturned in court when his legal team argued that his urine sample had not been handled according to protocol.
Other abused biological molecules include synthetic versions of human growth hormone and luteinizing hormone, which is involved in testosterone production. These hormones present challenges in detection to antidoping researchers. “Our job is always to differentiate between the naturally circulating substance from the doping agent. Whatever is identical to the human body is very difficult for us to identify and to prove that this substance was a misuse of a drug rather than a natural variation,” says biochemist Mario Thevis at the German Sport University. Most of the methods for detecting doping rely on chromatography and mass spectrometry.
Drugs available on the market aren’t the only headaches for antidoping authorities. A major component of the antidoping efforts is developing detection methods for pharmaceuticals not yet on the market. Thevis gives the example of selective androgen receptor modulators, which are a new type of anabolic agents in phase III clinical trials. They stimulate growth of muscle and bone. The potential drugs already are being abused, says Thevis, pointing to the case of Jamaican 400-meter runner Bobby-Gaye Wilkins, who was banned from the world championships in Doha in 2010 after she tested positive for a selective androgen receptor modulator called Andarine. That case, says Thevis, “demonstrated we are not chasing ghosts. We are going after drugs that are not yet approved for the market but are definitely being misused by elite athletes.”
The BALCO scandal of 2003 illustrated that some athletes were willing to take drugs that federal agencies and antidoping authorities didn’t even know existed. BALCO was a company that illicitly provided athletes with a substance known as “the clear” that was later identified by Catlin’s group to be the molecule tetrahydrogestrinone. THG is an anabolic steroid that binds to the androgen receptor to boost muscle mass. Federal law-enforcement authorities eventually caught a number of athletes who used it, including British 100-meter sprinter Dwain Chambers, American sprinter Marion Jones and MLB player Barry Bonds.
Blood and genetic tricks
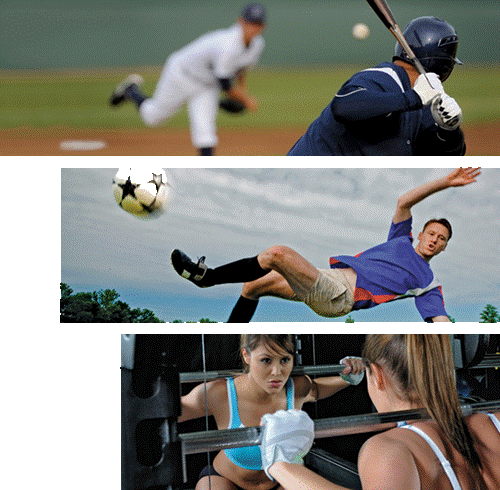
Blood doping is another matter: Cheating athletes get clinicians in their entourages to dupe the hemoglobin count test by adjusting their red blood cell counts before and after competition. In some cases, authorities track the volume percentage of red blood cells. In a tricky manipulation, cheaters increase their red blood cell counts by taking drugs like EPO during the off-season. They withdraw the hemoglobin-rich blood and refrigerate it. When competition season starts, once the antidoping inspector leaves with blood samples from the athletes for testing, the cheaters transfuse the stored blood back into their bodies and head out to compete loaded with extra hemoglobin. After the competition, they quickly withdraw some blood to bring their red blood cell count back to normal and wait for the antidoping inspector to do the post-competition test. Just as with some synthetic hormones, there isn’t a good way to tell apart stored red blood cells from ones that are currently circulating in the bloodstream to catch blood doping.
Genetic manipulations have been on WADA’s radar screen for a decade, although no athlete has yet been caught using them. Gene doping is essentially the flip side of gene therapy. Gene therapy has made recent gains in treating illnesses like genetic-based severe combined immunodeficiency disease and Leber congenital amaurosis, a retinal disease that progresses to total blindness by adulthood.
Because of gene doping’s growing potential, WADA’s science director, Olivier Rabin, says the agency has been proactive against its exploitation. “If an athlete were ever to be in a position to add an extra gene of EPO or growth factor, they can gain a significant advantage,” he says. “We believe that this technology one day will be an option for some athletes who will not hesitate to consider it.”
Gene doping is certainly attractive to manipulate muscle, blood and pain-perception systems –”anything that enhances the ability to train and to deliver blood to exercising tissues and to increase endurance or explosive muscle function,” says Theodore Friedmann at the University of California, San Diego, a gene therapy expert who helped to establish the organization’s research program in the area.
He says while gene doping isn’t yet reality, “there have been some high-profile instances of very prominent athletic trainers making attempts to obtain genetic tools, like the viral vectors that express transgenes.” Friedmann cites the 2006 case of a German trainer, Thomas Springsteen, who was arrested and brought to trial for making attempts to obtain Repoxygen, a drug that was in preclinical trials to put an erythropoietin gene into patients suffering from bone marrow failure from chronic kidney disease or cancer.
Always seeking new antidoping tools
Detecting gene and other forms of doping is complicated for authorities because they are limited in the types of samples they can take from athletes. They can’t do anything as invasive as muscle biopsies, so they have to rely on techniques that can detect doping in blood and urine. The experts cite the relatively new -omics methods as potential ways to better catch doping. “We believe they are going to be an important component of our arsenal,” says Rabin. “We have different projects looking at all the different levels of -omics.”
Rabin says WADA researchers have some interesting concepts in the works, such as looking for the molecular signatures of doping. “When an athlete dopes with a substance or a cocktail of substances, she or he is looking for a physiological impact. It’s to enhance transfer of oxygen, muscle mass and other different physiological capabilities,” says Rabin. “These create an imbalance in the body’s homeostasis that we believe will be reflected at different -omics levels.” He says a challenge researchers are facing is distinguishing between signatures caused by physical exertion, which elite athletes do intensely, and those signatures caused by doping.
For now, the thinking is that -omics technologies are not quite there yet for antidoping enforcement. Molecular geneticist James Rupert at the University of British Columbia in Canada received WADA funding to explore the possibility of using RNA transcripts to tell if an athlete has doped with EPO. “The test I was proposing would work for any source of erythropoietin, including gene doping,” he says.
But he says his group concluded after preliminary work in mice that there wasn’t sufficiently robust differential gene expression to be detected reliably in urine or blood. Rupert says there probably are differential gene expression patterns at the tissue level, but that doesn’t help antidoping authorities, because they cannot sample tissues. He adds that also compounding the problem is that the genetic variation and background frequencies of people cover a wide range. This is an impediment because “we really wouldn’t have any specificity for our test,” says Rupert. “It’s very important with a doping control test that you have high sensitivity and specificity, because you don’t want to falsely accuse people.”
To better track athletes and understand their individual physiologies, WADA implemented the Athlete Biological Passport program in 2009. The program tracks athletes throughout the year – not just at competitions – to make sure that they are not using performance-enhancing substances or methods. Antidoping authorities take measurements for blood, endocrine and steroid parameters to know what an athlete’s normal physiological range is and to find deviations.
The beauty of the biological passport, says Rupert, is that “you’re serving as your own control … Every time I measure your parameters, I reinforce what I know about you, so it makes my baseline values get better.” The moment an athlete deviates from his or her normal range, it’s a reason to be suspicious. Deviations can occur either because of doping or illnesses. Rupert suggests the biological passport should be expanded to use genetic information, which can help in some cases, such as the Eero Mäntyranta case , to tell genetic outliers from cheating athletes.
Real or fake ability?
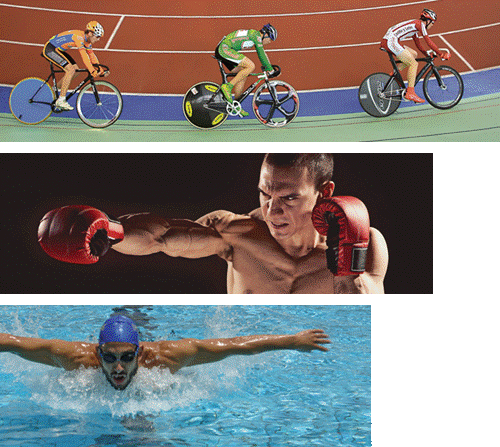
With the high stakes of money and fame in sports, doping will remain a fact in years to come. Athletes are desperate enough to try anything. They have scientists and clinicians in their support groups constantly mine the scientific literature, searching for clues about any substance that will improve performance and fly under the radar of the authorities. Even if a compound is shown to change properties of cells in a petri dish in a way that could be interpreted as better performance, some athletes are willing to give it a shot despite the lack of safety data.
“What is scary to us scientists is that some people are ready to take substances without proper clinical trials,” says Rabin. “I worked in the pharmaceutical industry [before]. We took all these precautions to go from animal models to first administrations in humans and then to patients. But then we realized some athletes didn’t even bother to wait. The drug went straight from the test tube to their bodies!”
Catching doping athletes is not just about bringing cheaters to courts of justice. Doping is dangerous and can be lethal. And performance enhancement doesn’t only affect the athletes involved: It cheats the fans. As Friedmann sums it up, “Are you watching an army of molecular biologists and chemists at work, or are you watching truly beautiful human effort?”
References
- Kresge, N. et al, J. Biol. Chem. 286, e2 – e3 (2011).
- Lasne, F.; de Ceaurriz, J; Nature405, 635 (2000).
- de la Chapelle, A. et al. Proc. Natl. Acad. Sci. USA90, 4495 – 4499 (1993).
- Klingmüller, U. et al. Cell, 80, 729 – 738 (1995).
- Jakobsson, J. et al. J. Clin. Endocrinol. Metab. 91, 687 – 693 (2006).
Enjoy reading ASBMB Today?
Become a member to receive the print edition monthly and the digital edition weekly.
Learn moreGet the latest from ASBMB Today
Enter your email address, and we’ll send you a weekly email with recent articles, interviews and more.
Latest in Science
Science highlights or most popular articles
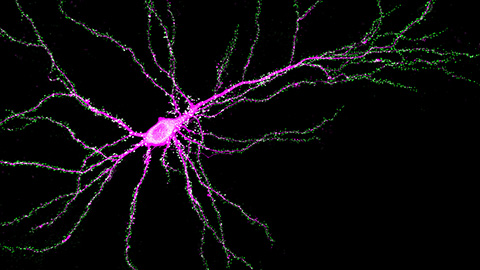
Scientists identify new function of learning and memory gene common to all mammalian brain cells
Findings in mice may steer search for therapies to treat brain developmental disorders in children with SYNGAP1 gene mutations.
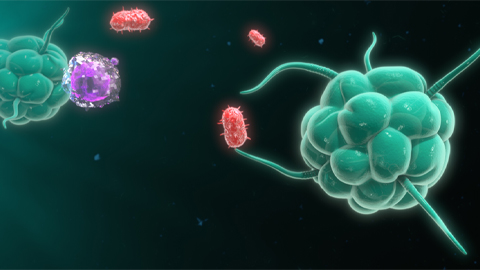
From the journals: JBC
Biased agonism of an immune receptor. A profile of missense mutations. Cartilage affects tissue aging. Read about these recent papers.
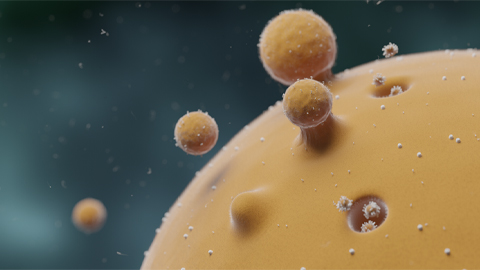
Cows offer clues to treat human infertility
Decoding the bovine reproductive cycle may help increase the success of human IVF treatments.
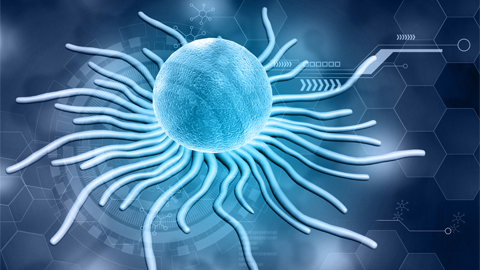
Immune cells can adapt to invading pathogens
A team of bioengineers studies how T cells decide whether to fight now or prepare for the next battle.
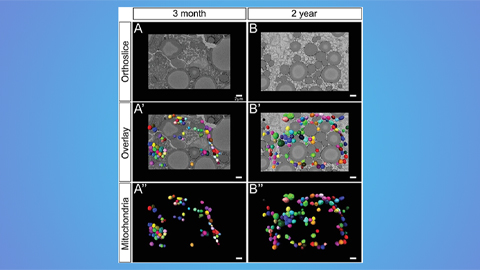
Hinton lab maps structure of mitochondria at different life stages
An international team determines the differences in the 3D morphology of mitochondria and cristae, their inner membrane folds, in brown adipose tissue.
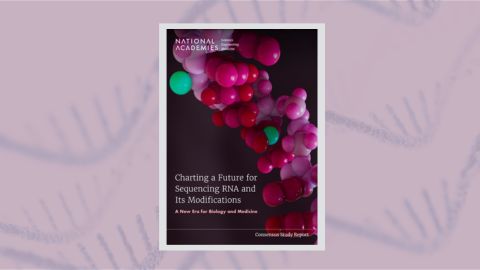
National Academies propose initiative to sequence all RNA molecules
Unlocking the epitranscriptome could transform health, medicine, agriculture, energy and national security.