JLR: A close-up of nascent HDL formation
Oil and water don’t mix. But our aqueous blood is full of hydrophobic lipids — including cholesterol. To travel via the bloodstream, those lipids must hitch a ride on an amphipathic carrier. In a paper in the Journal of Lipid Research, scientists at Boston University report an advance in our mechanistic understanding of how one such carrier forms.
“Lipoproteins are like boats that deliver and remove cargoes of fatty substances to and from our cells,” said David Atkinson, chair of the physiology and biophysics department at Boston University School of Medicine and senior author on the JLR paper.
The subset of those “boats” that carry cholesterol and other lipids to the liver from other parts of the body are called high-density lipoproteins, or HDL, aka “good cholesterol.” HDL can remove cholesterol from distal cells — such as macrophages in the walls of arteries, where cholesterol accumulation can lead to heart attacks — and deliver it to liver cells, a process known as reverse cholesterol transport. The liver disposes of excess cholesterol by converting it into bile acids secreted into the small intestine.
According to Atkinson, a biophysicist, most of what is known about HDL formation comes from experiments that take a cell biological tack. In such studies, he said, “You can see (HDL formation) happening, and you can quantitate what happens, but you don’t understand the driving interactions that cause it to happen.”
HDL is built on a scaffold protein, apolipoprotein A-I. This apoA-I is thought to collect cholesterol and phospholipids from the cell membrane. Atkinson’s team wanted to better understand that process.
A model of a high-density lipoprotein particle shows apolipoprotein A-I in pink, phospholipids in gray and cholesterol in yellow.Wu et al/JBC 2009ApoA-I depends on a lipid transporter protein, ABCA1, that pumps cholesterol from the inner to the outer leaflet of the cell membrane. Because the cholesterol that ABCA1 transfers usually ends up bound to apoA-I, some researchers suspected a physical interaction between apoA-I and ABCA1. Others argued that cholesterol and phospholipids could diffuse passively and bind to apoA-I.
“Even if you demonstrate that apoA-I binds to the cell surface, you don’t actually know that it’s bound to ABCA1. It’s just bound to the cell surface,” Atkinson said. So he asked his team to see if they could “demonstrate that interaction actually happening in the isolated components.”
The team, led by graduate student Minjing Liu and supported by Xiaohu Mei and Haya Herscovitz, used isolated apoA-I and ABCA1 to test for a physical interaction. They were able to show immunoprecipitation of apoA-I with purified ABCA1.
The lab earlier had designed a mutant apoA-I with a little extra wiggle in an already flexible hinge region. For this study, they used the mutant to show that higher flexibility increased apoA-I lipidation, or the formation of nascent HDL. The team has not yet tested whether the extra-flexible mutant binds to ABCA1 better or whether binding of either form of apoA-I activates ABCA1.
But about one thing Atkinson is certain: “It’s the apoA-I/ABCA1 interaction which then enables the nascent HDL particle formation to happen as the membrane components are being transported out by ABCA1.”
Increasing reverse cholesterol transport may be a way to reduce atherosclerosis and heart disease. Atkinson is optimistic about the promise of understanding the physiological processes better.
“Translational research might be in vogue,” he said, “but remember that if you don’t do foundational basic discovery research, you will not have anything to translate.”
Enjoy reading ASBMB Today?
Become a member to receive the print edition four times a year and the digital edition monthly.
Learn moreGet the latest from ASBMB Today
Enter your email address, and we’ll send you a weekly email with recent articles, interviews and more.
Latest in Science
Science highlights or most popular articles
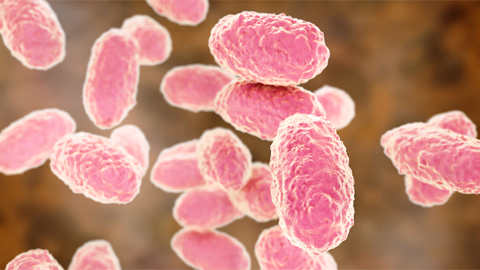
Targeting toxins to treat whooping cough
Scientists find that liver protein inhibits of pertussis toxin, offering a potential new treatment for bacterial respiratory disease. Read more about this recent study from the Journal of Lipid Research.
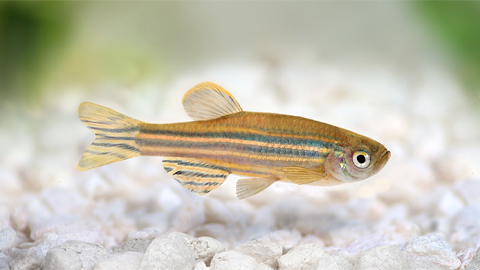
Elusive zebrafish enzyme in lipid secretion
Scientists discover that triacylglycerol synthesis enzyme drives lipoproteins secretion rather than lipid droplet storage. Read more about this recent study from the Journal of Lipid Research.
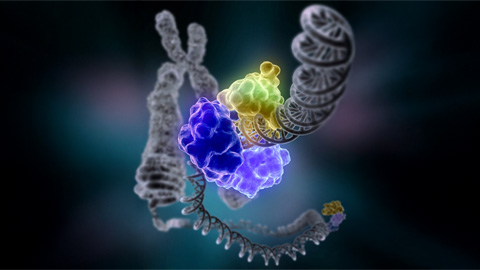
Scientists identify pan-cancer biomarkers
Researchers analyze protein and RNA data across 13 cancer types to find similarities that could improve cancer staging, prognosis and treatment strategies. Read about this recent article published in Molecular & Cellular Proteomics.
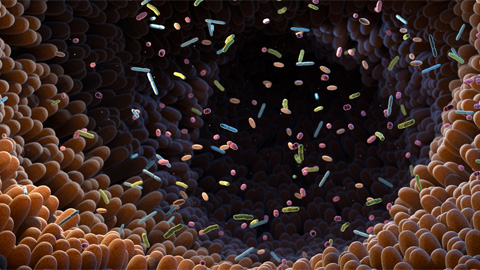
New mass spectrometry tool accurately identifies bacteria
Scientists develop a software tool to categorize microbe species and antibiotic resistance markers to aid clinical and environmental research. Read about this recent article published in Molecular & Cellular Proteomics.
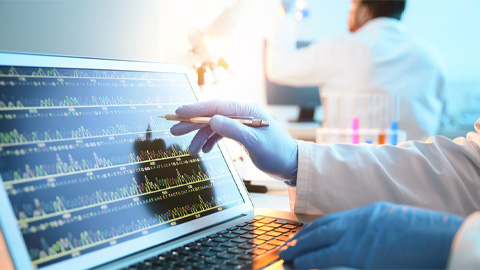
New tool matches microbial and metabolic metaproteomic data
Scientists develop a bioinformatics program that maps omics data to metabolic pathways. Read about this recent article published in Molecular & Cellular Proteomics
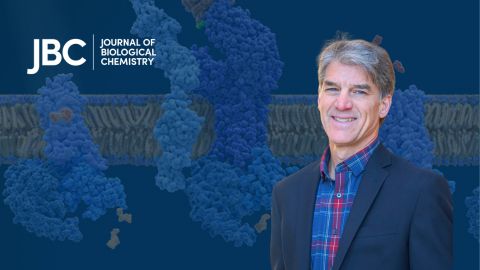
Meet Paul Shapiro
Learn how the JBC associate editor went from milking cows on a dairy farm to analyzing kinases in the lab.